Exposure Assessment Tools by Chemical Classes - Nanomaterials
Overview
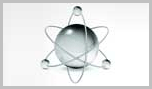
Nanoscale materials (or "nanomaterials") exhibit unique properties that can be defined by their small size, ≤100 nanometers in at least one dimension. While nanoscale materials often have the same molecular composition as larger, non-nanoscale materials, they represent a unique class of substances independent of their larger counterparts because the small size results in unique physical-chemical characteristics.
These distinctive characteristics allow for novel applications of intentionally engineered or manufactured nanomaterials, and can result in a unique set of fate, transport, exposure, and human or ecological health impact scenarios.
Illustration of size and scale of nanotechnology (Image courtesy of National Nanotechnology Initiative)
Types
Nanoscale materials can be grouped by their source: they can be naturally occurring, unintentionally produced, or specifically engineered.
- Naturally occurring nanomaterials are formed and released into the environment as a result of natural processes such as weathering/erosion and volcanic eruptions. These include compounds like amorphous silica, carbon black, and ultrafine particulate matter. Naturally occurring particulate matter includes mineral aerosols from wind erosion of soil, sea salt aerosols from wave sprays and evaporation, and ash and smoke particulates from forest fires.
- Unintentional, or incidental, nanomaterials include materials that are produced as a byproduct of an intentional anthropogenic process such as industrial processing, laboratory procedures, and combustion. An example of an incidental nanomaterial is ultrafine particulate matter from automobile exhaust.
- Engineered nanomaterials include a wide range of compounds that are intentionally manufactured in a research or commercial setting. They are designed to exhibit specific properties and maintain uniform structures, so that they can be used in a wide range of advanced applications. There are many different chemical compositions of engineered nanoscale materials, each with unique physical-chemical properties. Nanomaterials have existed for millennia - silver, gold, and carbon nanomaterials were unknowingly manipulated to create lustrous pottery and jewelry, vibrant stained glass, and resilient swords as far back as the 4th Century.
However, modern nanotechnology, which involves understanding and controlling nanoscale materials through imaging, measuring, modeling, and manipulating matter, is a relatively new and rapidly growing field. Many modern nanomaterials and novel applications are still being developed and research into their associated effects, exposure, and risks is ongoing.
The focus of this module is on exposure assessment of engineered nanomaterials because their small size and unique physical and chemical properties might affect how people are exposed. Exposure to other incidental or naturally occurring nanomaterials are discussed in other modules focused on general exposure assessment.
While there are no set categories or classification methods currently used to group engineered nanomaterials, there are distinct groups that exist based on the chemical composition and physical arrangement of the material - such as carbon-based nanotubes and nanofibers, metal or metal-oxide nanoparticles, and selenide-based quantum dots.
The International Organization for Standardization (ISO) has published a standard (ISO/TS 27687:2008) that lists terms and definitions related to particles in the field of nanotechnologies. Major groups of engineered nanomaterials are described in more detail below.
Major Groups of Engineered Nanomaterials Based on Chemical Composition and Physical Structure | |
---|---|
Carbon-based Nanomaterials are molecules composed primarily of carbon; intentionally organized in a specific shape or form such as a hollow sphere, ellipsoid, or tube; and possess unique strength, conductivity, and thermal properties. While carbon-based nanomaterials contain only carbon, modified forms of carbon-based nanomaterials may contain surface groups with non-carbon atoms, or could be engineered to surround or encapsulate a non-carbon atom, such as a metal.
|
![]() (Image source: Wiki Commons) |
Dendrimers are repetitively branched molecules - typically symmetrical and spherical - which provide internal cavities for other molecules and possess unique surface functionality.
|
![]() (Image Source: Wiki Commons) |
Metal-/Metal Oxide-Based Nanomaterials are generally spherical nanoscale particles composed entirely or partly of one or more metals; they possess unique optical, thermal, magnetic, conductivity, and oxidative/reductive properties.
|
![]() (Image source: Wiki Commons) |
Quantum Dots are nanocrystalline semiconductors (usually metal complexes, selenides, or sulfides) with unique optical and electrical properties. For example, manipulating the size of quantum dot particles in solution will result in different fluorescent colors.
|
![]() (Image courtesy of National Nanotechnology Initiative ) |
Exposure and Hazard Considerations for Nanoscale Materials
- Unique absorption and distribution patterns due to small size (e.g., can cross cell membranes more readily through use of transport structures meant for biological molecules, such as ion channels; are similar size to proteins so can be confused by the body).
- Surface chemistry - many nanomaterials have highly reactive OR highly non-reactive surface chemistries; meaning they will interact uniquely with other molecules in the body compared with materials larger than nanoscale. Surface chemistry is often complex and is a driving factor of how the molecule behaves (e.g., transport/fate).
- Surface-to-volume ratio - nanomaterials can have a much higher surface area to volume ratio compared to the same mass of a non-nanoscale-sized material, so they can be more reactive on a mass-to-mass basis. As a result, many nanomaterials may be more toxic than non-nanoscale-sized materials on a mass-to-mass basis.
Physical/Chemical Characteristics
A defining characteristic of nanomaterials is their size; nanomaterials are typically defined as particles or other substances that are less than 100 nanometers in at least one dimension.
The small size of nanomaterials influences the way that these substances behave in the environment because of quantum effects and surface-to-volume ratios. Two important aspects of the behavior and characteristics of small particles that affect exposures are described here.
- Physics of Small Particles. At the nano scale, "quantum effects" dictate the physical and chemical properties of the material, and these properties can be very different than those of the non-nanoscale-sized material. Quantum mechanics describe the physics behind particle and atom interactions.
- An example of quantum mechanics resulting in unique characteristics of nanomaterials is nanoscale gold. Unlike non-nanoscale-sized gold, which interacts with light to create the radiant yellow color it is prized for, nanoscale gold appears red or purple. The nanoscale size translates to unique optical properties because the movement of the gold electrons are restricted, and therefore interactions with light change.
- Other unique properties that can result from quantum effects include enhanced reactivity, improved catalysis, tunable wavelength sensing ability, increased mechanical strength, electrical conductivity, and stronger oxidative and reductive potential.
- An example of quantum mechanics resulting in unique characteristics of nanomaterials is nanoscale gold. Unlike non-nanoscale-sized gold, which interacts with light to create the radiant yellow color it is prized for, nanoscale gold appears red or purple. The nanoscale size translates to unique optical properties because the movement of the gold electrons are restricted, and therefore interactions with light change.
- Surface-to-Volume Ratio. In addition to quantum mechanics, a high surface-to-volume ratio influences nanomaterial behavior, resulting in a proportionally greater amount of the material at the "surface" to interact with the surrounding environment. (The image below illustrates this concept.) Transport, deposition, and uptake mechanisms which rely on surface interactions and size factors will therefore differ compared to larger compounds.
The surface of a nanomaterial is also important because many engineered nanomaterials can be “functionalized”, meaning they are altered in a way that changes reactivity with other molecules or interaction with the environment - for example, through the addition of reactive surface structures such as amine groups or carboxyl groups.
Illustration of how surface area-to-volume ratio of nanomaterials is increased compared with non nanoscale-sized materials. (Image courtesy of National Nanotechnology Initiative)
In addition to the physics of small size particles and high surface-to-volume ratio of nanomaterials, it is also important to consider the following when characterizing exposure:
- Shape of the nanoscale material
- Surface area and surface-to-volume and/or surface-to-mass ratios of individual particles
- Distribution and agglomeration (bundling) of individual nanoscale particles
- Surface functionalization (i.e., presence of other substances bound to the surface of a nanoparticle) and the resulting changes in both chemistry and surface topography
- Impurities within a solution
- State (solid, liquid, or gas) and dispersion/suspension in media
- Hydrophobicity and solubility
- Dissolution kinetics
Routes
Exposure to nanomaterials can occur through inhalation, ingestion, or dermal absorption. Further, biomedical uses can result in exposure via injections. The most likely routes differ greatly depending on the individual nanomaterial’s physical-chemical properties (and how these properties impact fate between environmental media) as well as the application in which the material is used.
The National Nanotechnology Initiative Workshop on Human and Environmental Exposure Assessment (2009) came to several broad conclusions on exposure routes, including:
- Exposure routes and scenarios will depend on the use of the nanomaterial
- All routes may need to be considered, due to the wide variety of nanomaterial uses
- "Dermal exposure data should be included in any survey," as more research is needed regarding possible skin absorption of nanomaterials
- Both intended use and misuse of consumer products can lead to exposure, and many key aspects of exposure scenarios are not well understood
Researchers must consider how the physical-chemical properties of a specific nanomaterial impact fate between environment media, and how the application of a nanomaterial results in different exposure scenarios. Different nanomaterial applications can result in distinct primary exposure routes.
For example, the use of nano-Ag in disinfectant spray could result in a primary exposure route of inhalation, whereas nano-Ag in a coating on a keyboard could result in primarily dermal exposure.
Material and product manufacturing processes as well as research and development efforts can result in unique occupational exposure scenarios following inhalation, ingestion, or dermal absorption routes. Furthermore, material and product manufacturing and product use, re-use, and recycling can result in numerous secondary exposure pathways that end with ingestion, inhalation, or dermal absorption.
Dermal Absorption |
---|
Dermal absorption of nanomaterials might be greater than for other products due to their small size: many nanoparticles are small enough that they are suspected to easily cross the skin barrier—though, further research is needed on this topic. Initial evidence suggests that nanomaterials will not pass through the dermal layer, but surface coatings or other modifications could facilitate absorption from this exposure route (Crosera et al., 2009). |
Examples of applications of nanomaterials which may lead to exposure via dermal routes include:
|
Inhalation |
---|
Some nanomaterials are intentionally aerosolized, as they are used in spray products or applied to other products during manufacturing using spray-dispersion techniques. Other nanomaterials are unintentionally aerosolized during different manufacturing or production activities, disposal, or recycling. Depending on their physical-chemical properties, aerosolized nanomaterials may remain dispersed in ambient or indoor air where they can be inhaled, or the nanomaterials may aggregate/agglomerate with each other or sorb to dust. Nanomaterials that aggregate/agglomerate or sorb to dust are likely to settle out of the air, making inhalation a less relevant route of exposure. Conversely, aerosolized nanomaterials that are inhaled could penetrate deeper into the lung than their non-nanoscale counterparts, resulting in differences in exposure along the respiratory tract. |
Examples of applications of engineered nanomaterials which may lead to exposure through inhalation include:
|
Ingestion |
---|
Many pharmaceutical and biomedical applications of nanomaterials exist, and some of these applications result in direct ingestion of a nanomaterial. Additionally, nanomaterials released into the air or released from numerous consumer products or food packaging may sorb to dust particles or settle onto food. Similar to dermal and inhalation routes, the small size of nanomaterials may result in different absorption patterns following ingestion compared to their non-nanoscale counterparts. |
Examples of applications of engineered nanomaterials which may lead to exposure through ingestion include:
|
Media
Due to the wide range of nanomaterial compositions and applications, it is theoretically possible for nanomaterials to be found in any environmental media, including indoor and outdoor air, surface water and drinking water, soil, sediment, and indoor dust.
Research questions laid out by the National Nanotechnology Initiative Workshop on Human and Environmental Exposure Assessment (NSET 2011) and Workshop on Nanomaterials and the Environment and Instrumentation, Metrology, and Analytical Techniques (NSET 2011) regarding behavior of nanomaterials in various environmental media include:
- Will nanomaterials in air follow conventional particle dispersion models? How do the small particle size and aggregation properties of specific nanomaterials affect particle behavior?
- Will nanomaterials in water aggregate and settle like many non-nanoscale-sized suspended solids, or will they remain dispersed in the water column? How do the water qualities, such as pH or presence of natural organic matter, impact aggregation of nanomaterials in water?
- Are detection/characterization methods that exist for non-nanoscale-sized materials, which typically are mass-based, appropriate for nanomaterials which are unique for their qualities at small mass?
Additionally, the NNI Workshop Reports outlined many challenges that currently exist for detecting, characterizing, and quantifying nanomaterials in environmental media, including:
- The physical-chemical properties of an engineered nanomaterial can be altered or modified by the environment (e.g., surface chemistry of carbon nanotubes can be altered following UV exposure; physical abrasion can result in shortening of nanofibers), making characterization difficult.
- Little data are available regarding the effect of variables such as sample preparation techniques, storage temperatures, and solvent interactions on detection/quantification methods; therefore, comparing results across studies with different protocols is difficult.
- Many methods for quantification and analysis have not been validated, meaning issues of quality control, sensitivity, reliability, and reproducibility exist around most current studies measuring environmental concentrations of nanomaterials (or predicting based on behavior in controlled laboratory settings).
Recent reports from NRC and OECD also lay out research questions related to exposure to nanomaterials (NRC, 2012; OECD, 2012).
Exposed Populations
Currently, many engineered nanomaterials and nanomaterial applications are still in the research and development stage. Therefore, workers involved with these fields represent a key population that may be at high risk for exposure.
The use of nanoscale materials in consumer products is increasing rapidly. According to the Project on Emerging Nanotechnologies (PEN) nanomaterials are found in electronics, cosmetics, automotive, and medical products. PEN maintains a searchable inventory of “nano” products that includes manufacturer-identified consumer products currently on the market.
While nanomaterials in electronics have limited exposure potential for consumers (as the nanomaterial is usually embedded in the product interior), individuals who work in electronic manufacturing or electronic recycling may be occupationally exposed to nanomaterials. Other nano-enabled products may result in higher exposures to the consumer, such as cosmetics containing nanomaterials.
Nanomaterials are also currently used many biomedical devices, such as imaging technologies or drug treatments. Biomedical engineers and pharmaceutical scientists may experience occupational exposure during the development and manufacturing of these applications, while medical professionals and patients may incur exposure through their use.
Tools
References
Crosera, M; Bovenzi, M; Maina, G; Adami, G; Zanette, C; Florio, C; Filon Larese, F. (2009). Nanoparticle Dermal Absorption and Toxicity: A Review of the Literature [Review] Exit. Int Arch Occup Environ Health 82: 1043-1055.
National Nanotechnology Initiative Workshop on Human and Environmental Exposure Assessment (2009) (PDF) (92 pp, 2.32 MB, About PDF) Exit
NRC (National Research Council). (2012). A Research Strategy for Environmental, Health, and Safety Aspects of Engineered Nanomaterials Exit. Washington, DC: National Academies Press.
NSET (Nanoscale Science, Engineering, and Technology). (2011). Human and Environmental Exposure Assessment: Report of the National Nanotechnology Initiative Workshop, February 24-25, 2009 (PDF)(92 pp, 2.32 MB, About PDF) Exit: Part I of IV in the 2009-2010 NNI Environmental, Health, and Safety Workshop Series. Committee on Technology of the National Science and Technology Council (NSTC), Nanoscale Science, Engineering, and Technology (NSET) Subcommittee.
NSET (Nanoscale Science, Engineering, and Technology). (2011). Nanomaterials and Human Health & Instrumentation, Metrology, and Analytical Methods (PDF)(76 pp, 772 K, About PDF): Report of the National Nanotechnology Initiative Workshop, November 17-18, 2009: Part III of IV in the 2009-2010 NNI Environmental, Health, and Safety Workshop Series. Committee on Technology of the National Science and Technology Council (NSTC), Nanoscale Science, Engineering, and Technology (NSET) Subcommittee.
OECD (Organisation for Economic Co-operation and Development). (2012). Important Issues on Risk Assessment of Manufactured Nanomaterials. In Series on the Safety of Manufactured Nanomaterials, No 33 Exit. (ENV/JM/MONO(2012)8). Paris.
PEN (The Project on Emerging Nanotechnologies). (2015). The Project on Emerging Nanotechnologies Exit (accessed 2015).