Exposure Assessment Tools by Chemical Classes - Other Organics
Overview
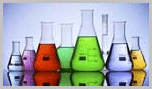
Organic compounds are generally defined as substances with structures based on carbon. Although some carbon-based substances are classified as inorganic, all substances classified as organic are carbon-based. Organic compounds comprise a highly variable group of ubiquitous substances with uses ranging from consumer products (e.g., ingredients in personal care products, building materials, food packaging, clothing, furniture) and drugs (e.g., Ibuprofen) to fuel components (e.g., hydrocarbons) and explosives (e.g., TNT). Many pesticides are also classified as organic – these are discussed in more detail in the Pesticides Module in the Chemical Classes Tool Set of EPA ExpoBox.
Organic compounds can be natural, synthetic, or semi-synthetic. The source and method by which organic compounds are produced can affect their properties and expected exposure scenarios. For example, many natural organic compounds, such as lipids, proteins, and carbohydrates, are plant-based and have medicinal or nutritional benefits that are exploited through direct exposure to these compounds.
On the other hand, some synthetic organic compounds are produced unintentionally, such as combustion byproducts like polycyclic aromatic hydrocarbons (PAHs) and dioxin released from industrial chlorinated processes, and exposures to these compounds might be largely indirect.
Organic compounds are often grouped according to structural similarities. Different “classes” of organic compounds refer to groups based on specific structural characteristics, including chemical bonds and functional groups. Similarities in chemical structure affect the properties of organic compounds, and thus their uses and human exposure considerations.
The environmental fate, transport, and exposure of organic compounds can be estimated based on models that take into account certain physicochemical properties that can help predict partitioning behavior in the environment. Some physicochemical properties are also important to consider when designing appropriate analytical methods for classes of organic compounds.
The behavior of organic compounds varies widely; however, some generalities can be made for subgroups of compounds. The behavior or organic compounds in the environment is affected by the physicochemical properties of the compound, including molecular weight and the presence of specific functional groups, such as alcohols, amines, carboxylic acids, and ketones.
These properties and characteristics often affect a compound’s solubility, partitioning behavior, and reactivity in relatively predictable ways, allowing exposure assessors to easily conduct screening-level assessments to estimate which organic compounds might be of highest concern.
It is not the intent of this tool set to provide information relevant to all organic compounds. As mentioned above, organics comprise a highly variable group of substances with a large range of uses. Instead, this tool set focuses on tools for assessing exposures to five specific groups of organic compounds of concern, including:
- Dioxins, Furans, and PCBs
- Flame Retardants
- Hydrocarbons
- Perfluorinated Compounds (PFCs)
- Phthalates and Bisphenol A (BPA)
These compound groups are known to possess properties that increase the potential for human and ecological exposure and are addressed specifically in this tool set because of their relevance to developing policy and risk management efforts.
Many organic compounds identified above fall into a category known as persistent organic pollutantspersistent organic pollutantsPersistent Organic Pollutants (POPs) are chemical substances that persist in the environment, bioaccumulate through the food web, and pose a risk of causing adverse effects to human health and the environment. United Nations Environment Programme (https://www.chem.unep.ch/pops/), or POPs, including PCBs, dioxins, furans, brominated flame retardants, and perfluorinated compounds (PFCs). These compounds have long half-lives in environmental media and are subject to long-range transport through the environment, meaning that exposure can occur long after the chemical is released and at locations far from the original source of the contaminant.
Many persistent organic compounds also have a tendency to accumulate in biota, meaning that significant secondary exposures can occur through ingestion of foods from higher levels of the food chain, like fish, meat, and dairy products. Information specific to POPs is also provided in this tool set.
Volatile Organic Compounds, or VOCs, are another category that overlaps with the other groups described in this tool set. Similar to POPs, VOCs are defined by a physicochemical property that imparts unique fate and transport and exposure considerations - high vapor pressure at room temperature. Because of their high volatility, VOCs are common air pollutants in both outdoor and indoor environments.
VOCs can be further categorized based on boiling point as very volatile organic compounds (VVOCsVVOCsFor example, propane and butane. Boiling point between <0 to 50–100 °C. See reference: https://19january2021snapshot.epa.gov/iaq/voc2.html#classifications) and semi volatile organic compounds (SVOCs
SVOCsFor example, DDT, phthalates, and fire retardants. Boiling point between 240–260 to 380–400 °C. See reference: https://19january2021snapshot.epa.gov/iaq/voc2.html#classifications). Several substances including DDT, chlordane, and PCBs are classified as both SVOCs and POPs. Information specific to VOCs is also provided in this module.
PCB and dioxin resources can be found here:
Polychlorinated Biphenyls (PCBs)
Dioxin
Resources related to hydrocarbons and specifically PAHs are also available.
Technology Transfer Network - Polycyclic Organic Matter (POM)
Toxic Substances Portal - Polycyclic Aromatic Hydrocarbons (PAHs)
National Biomonitoring Program - Polycyclic Aromatic Hydrocarbons (PAHs)
Dioxins, Furans, and PCBs
Polychlorinated dibenzo dioxins (PCDDs) and furans (PCDFs) and polychlorinated biphenyls (PCBs) are examples of persistent organic pollutant (POPs).
Dioxins, furans, and PCBs share a similar base structure of two 6-carbon rings with variable chlorine substituents. The term "dioxin" can be somewhat ambiguous, however, because the term is used to describe various groups of chemicals as well as one chemical, in particular. "Dioxins" generally refer to a group of seven PCDDs, ten PCDFs, and twelve "dioxin-like" PCBs, which are grouped together based on similar structural characteristics and mechanisms of action. The specific PCDD congener 2,3,7,8-TCDD is the most widely studied dioxin and is therefore often referred to simply as "dioxin".
- Learn more at Dioxin
PCDDs and PCDFs are not commercially produced or manufactured chemicals; rather, they are released into the environment as a by-product of combustion (burning) or other industrial processes. PCBs, on the other hand, were manufactured until 1979 for use in numerous commercial and industrial applications. The flame-retardant and insulating properties of these compounds were exploited for use in transformers, capacitors, cable insulation and other electrical equipment, hydraulic systems, plastics, paints, and carbonless copy paper, among other applications. Before they were banned, PCBs were released into the environment during manufacture and use and are still released into the environment through poorly maintained hazardous waste sites or leaks from old equipment.
Physicochemical Properties
PCDDs, PCDFs, and PCBs are persistent, halogenated, aromatic hydrocarbons characterized by a basic structure of two joined, chlorinated, 6-carbon rings. PCBs have anywhere from 2 to 10 chlorine atoms bonded to the total 12 carbon atoms, resulting in 209 different congeners. PCDDs and PCDFs are different from PCBs in that the two carbon rings are joined together by oxygen atoms, creating a three - ring structure. Chlorine atoms bond to the remaining available carbons to create 75 distinct PCDD congeners and 135 PCDF congeners. Of the 75 PCDDs, 7 of them (including 2,3,7,8-TCDD) are especially toxic.
Ten of the 135 PCDF congeners and 12 of the 209 PCBs have "dioxin-like" properties. The number and configuration of the chlorine compounds play a large role in determining properties of each PCDD, PCDF, and PCB congener. For example, melting point and lipophilicity increase with increasing degree of chlorination and vapor pressure and water solubility decrease.
Examples of Organochlorine Structures: Dioxins, Furans, and PCBs | ||
---|---|---|
PCDDs![]() |
PCDFs![]() |
PCBs![]() |
The table below provides a summary of key physicochemical factors that are likely to affect partitioning and fate of dioxins, furans, and PCBs in the environment. For chemical-specific values, consult the resources provided in the introduction to this module.
Property | Fate and Transport Implications |
---|---|
Dioxins and Furans | |
Vapor pressure at 25°C (atm) |
Low vapor pressure indicates that PCDDs and PCDFs will not readily volatilize from the pure organic state. |
Henry’s Law Constant |
Henry’s Law Constants indicate that volatilization of PCDDs and PCDFs from water to air might be a significant transfer mechanism during warmer temperatures, which could result in seasonal volatilization/deposition and long-range air transport. |
Solubility in water (mg/L) |
Low water solubility indicates that PCDDs and PCDFs will not readily dissolve in water. |
Octanol-Water Partition Coefficient (log value) |
Moderate-high log octanol-water partition coefficients indicate that PCDDs and PCDFs will preferentially partition to organic matter in soils and sediments, bioaccumulate, and biomagnify in aquatic ecosystems and possibly in terrestrial ecosystems and humans. |
Octanol-Air Partition Coefficient (log value) |
High log octanol-air partition coefficients indicate that PCDDs and PCDFs will preferentially partition to organic substances from air and bioaccumulate and biomagnify in terrestrial ecosystems and humans. |
Summary: Based on the physicochemical properties of PCDDs and PCDFs, these compounds are expected to accumulate in soils, sediments, and both aquatic and terrestrial biota. These substances might be driven to escape from water, which will lead to sorption to organic matter and volatilization to air, particularly during warmer weather. The expected seasonal volatilization/deposition cycle of these chemicals is expected to result in long-range transport in air and ultimate deposition at the poles. | |
PCBs | |
Vapor pressure at 25°C (atm) |
Low to moderate vapor pressure indicates that some PCBs will not readily volatilize from the pure organic state, while others are expected to be semi-volatile. |
Henry’s Law Constant |
Henry’s Law Constants indicate that volatilization of PCBs from water to air is expected to be a significant transfer mechanism which could result in seasonal volatilization/deposition and long-range air transport. |
Solubility in water (mg/L) |
Low water solubility indicates that PCBs will not readily dissolve in water. |
Octanol-Water Partition Coefficient (log value) |
Moderate log octanol-water partition coefficients indicate that PCBs will preferentially partition to organic matter in soils and sediments, bioaccumulate, and biomagnify in aquatic ecosystems and possibly in terrestrial ecosystems and humans. |
Octanol-Air Partition Coefficient (log value) |
Moderate-high log octanol-air partition coefficients indicate that PCDDs and PCDFs will preferentially partition to organic substances from air and bioaccumulate and biomagnify in terrestrial ecosystems and humans. |
Summary: Based on the physicochemical properties of PCBs, these compounds are expected to accumulate in soils, sediments, and aquatic and terrestrial biota. These substances might be driven to escape from water and volatilize from the pure organic form, which could result in accumulation in air and long-range transport, particularly of semivolatile PCBs. The expected seasonal volatilization/deposition cycle of these compounds is expected to result in ultimate deposition at the poles. |
Routes
Dioxins and PCBs are ubiquitous in the environment and partition readily to the fatty tissue of animals, resulting in accumulation in the food chain. Humans are primarily exposed to these compounds through ingestion of contaminated foods such as meat, dairy, fish, and shellfish. In utero exposure and exposure due to ingestion of breastmilk also occur and are exposure pathways of concern.
For PCBs that are semivolatile, inhalation exposure is also possible. However, the relatively low volatility of dioxins, furans, and some PCBs under typical environmental conditions suggests that direct inhalation might be a less significant route of exposure.
Note: The Routes Tool Set of EPA ExpoBox provides additional information and resources organized by route.
Media
Dioxins, furans, and PCBs are often found in sediment and soils. They are slow to degrade and tend to accumulate in living organisms, so can be found in biota and food, particularly dairy, meat, and fish products.
Media | Sources of Dioxins, Furans, and PCBs |
---|---|
Air |
|
Water |
|
Soil |
|
Food |
|
Aquatic Biota |
|
Consumer Products |
|
Note: The Media Tool Set of EPA ExpoBox provides additional information and resources organized by media.
Exposed Populations
Background exposure to ubiquitous dioxin and PCB mixtures results in body burdens of these compounds in all humans. The general population is further exposed to dioxins and PCBs through ingestion of contaminated food. Specific populations might experience increased exposures to the compounds due to lifestage, geographical location, or behavior.
These populations include developing fetuses, breast-feeding infants, children, human and ecological populations living in certain regions, and certain occupational workers.
- Long range atmospheric transport can result in higher environmental contamination levels in certain regions of the globe; people who live in these regions may have higher levels of exposure.
- The lipophilic nature of dioxins and PCBs contributes to the accumulation of these chemicals in breast milk. Infants who are breastfed may be exposed to the chemicals through ingestion.
- Individuals who consume high amounts of fish or aquatic mammals (i.e., seal, whale), may be exposed to dioxins and PCBs, particularly if the fish is sourced from contaminated waters. Fatty cuts of beef or other meats may also contain measureable dioxin or PCB contamination.
- Certain occupations may result in higher exposure levels to workers.
- PCB-waste is often labeled as industrial waste and treated as hazardous material. People who live near or work at hazardous waste landfills or hazardous waste incineration sites with PCBs may be at risk for higher exposure, especially if proper controls are not in place (ATSDR, 2000).
Note: The Lifestages and Populations Tool Set of EPA ExpoBox has addition information about particular population groups and lifestages.
Tools
Flame Retardants
Flame retardants are chemicals or other manufactured materials that confer qualities that resist or inhibit the spread of fire. Although some flame-retardant organic compounds have been banned or voluntarily phased out by manufacturers due to health concerns (e.g., many polybrominated diphenyl ethers [PBDEs] and polychlorinated biphenyls [PCBs]), these compounds may persist in the environment.
A wide range of organic chemicals are used in flame-retardant products, but most can be classified as halogenated (i.e., functionalized with bromine or chlorine), phosphorous-based, nitrogen-based, or as a combination of the aforementioned components. Two principal processes are used for incorporating flame-retardant materials into products: reactive and additive.
Whereas the reactive processes produce covalent bonds between the flame retardant and a polymer matrix that effectively lock most of the flame-retardant chemical in the product, the additive processes do not form such bonds and therefore increase the potential for the flame-retardant chemical to leach out of the product to which it is applied.
One of the principle mechanisms by which flame-retardant chemicals (particularly of the halogenated variety) confer flame resistance is through gas-phase radical quenching in which the flame-retardant material thermally degrades and releases chemical radicals that are highly reactive with oxygen, thereby reduces the amount of free oxygen available to supply the combustion process.
The result of this process is the release of some of the halogen atoms from the flame-retardant chemical and the transformation of the chemical from highly halogenated substances to a lower-halogenated congener.
Example structures selected from the range of traditional and emerging flame-retardant organic compounds are provided below to demonstrate the diversity of compounds in this group.
Example Flame Retardants | |
---|---|
Polybrominated Diphenyl Ether (PBDE)![]() |
Tetrabromobisphenol A (TBBPA)![]() |
Tetrabromophthalate (TBPH)![]() |
Tetrabromobenzoate (TBB)![]() |
Hexabromocyclododecane (HBCD)![]() |
Melamine![]() |
Chlorinated Paraffins![]() |
Tris (1,3-dichloro-2-propyl) Phosphate (Chlorinated Tris or TDCPP) ![]() |
Tributyl Phosphate![]() |
Triphenyl Phosphate (TPP)![]() |
Physicochemical Properties
The table below provides a summary of key physicochemical factors that are likely to affect partitioning and fate of select flame retardants in the environment. For chemical-specific values, consult the resources provided in the Chemical Classes Overview.
Property | Fate and Transport Implications |
---|---|
Halogenated (brominated) Flame Retardants | |
Vapor pressure at 25°C (atm) |
Low vapor pressure indicates that BFRs will not readily volatilize from the pure organic state. Vapor pressure decreases with increased degree of halogenation. |
Henry’s Law Constant |
The Henry’s Law Constant of BFRs corroborates with the low vapor pressure, indicating low volatility. |
Solubility in water (mg/L) |
BFRs generally have low water solubility, meaning they are not likely to dissolve in surface water. |
Octanol-Water Partition Coefficient (log value) |
The high Kow values of BFRs indicate high potential to bioaccumulate. |
Octanol-Air Partition Coefficient (log value) |
High log Koa indicates strong propensity of the compounds to absorb to organic matter such as soil particles, dust, or vegetation rather than remain in air (U.S. EPA, 2010). |
Summary: Traditional brominated flame retardants are highly persistent in soil and sediment, and will sorb to organic matter in the water column or air (i.e., dust). These compounds have a high potential for bioaccumulation. | |
Phosphorous-based Flame Retardants (PFRs) | |
Vapor pressure at 25°C (atm) |
Low vapor pressure indicates that PFRs will not readily volatilize from the pure organic state, where they exist as liquids. If aerosolized, PFRs are likely to exist in the vapor phase. |
Henry’s Law Constant |
While vapor pressure indicates volatilization from dry soils is unlikely, the moderate Henry’s Law constants of most PFRs indicate that volatilization from moist soil may occur for some PFRs. |
Solubility in water (mg/L) |
Relatively low water solubility of most PFRs indicates that they will not readily dissolve in water and have high soil adsorption potential. |
Octanol-Water Partition Coefficient (log value) |
While log Kow values for PFRs vary greatly, most PFRs have positive log Kow values, suggesting that these compounds will preferentially partition to organic matter in soils and sediments, and some could bioaccumulate and biomagnify in aquatic ecosystems and possibly in terrestrial ecosystems and humans. |
Octanol-Air Partition Coefficient (log value) |
While Koa values have not been identified, vapor pressure indicates that transfers from organic media (i.e., soil) to air are possible. |
Summary: Properties of the different PFRs vary greatly. PFRs are generally poorly soluble and lipophilic and will partition to soil, sediment, or organic matter rather than remain in water or air. However, most PFRs are less lipophilic than the brominated FRs and there is wide variation in Henry’s Law Constants and vapor pressure which indicates that the PFRs may occur in any type of media (van der Veen and de Boer, 2012). |
Routes
Exposure to flame retardants occurs mainly through inhalation or ingestion of dust. Food and water contaminated with flame retardants is another source of exposure. Exposure from dermal contact with contaminated soil and dust may also occur.
Route | Potential Sources of Flame Retardant Exposure |
---|---|
Inhalation |
|
Ingestion |
|
Dermal Contact |
|
Note: The Routes Tool Set of EPA ExpoBox provides additional information and resources organized by route.
Media
PBDEs have been measured in indoor and outdoor air, water and sediment, and soil. They have also been found in household dust. Flame retardants are widely used in consumer products, making indoor environments particularly important for this group of compounds.
Exposed Populations
Flame retardants are ubiquitous environmental contaminants, resulting in potetnial exposure across the general population. Certain populations may be at risk for higher exposures, for example:
- Children may be exposed to high levels of dust in homes due to the amount of time they spend on the floor and the higher level of hand-to-mouth contact. Additionally, many consumer products intended for children are intentionally treated with flame retardants, including pajamas, changing pads, and car seats.
- Workers involved with product manufacturing and product end-of-life processes (recycling, incineration, landfilling) may be exposed to flame retardant chemicals that are applied to numerous consumer products.
- Firefighters and other emergency personnel who may respond to home, business, or vehicular fires could be at risk for high exposures as flame retardants are released as materials succumb to fire.
Note: The Lifestages and Populations Tool Set of EPA ExpoBox includes addtional information about particular population groups and lifestages.
Tools
Hydrocarbons
Hydrocarbons contain only hydrogen and carbon atom in various configurations, including chains, branched chains, and rings. Most hydrocarbons fall into one of the five structural categories described in the table below.
Subclass | Description | Example Structures | |
---|---|---|---|
n-Alkanes | Saturated, unbranched, hydrocarbon chains | Methane, CH4![]() |
n-Hexane, C6H14![]() |
Branched alkanes | Saturated, branched, hydrocarbon chains | Isobutane, C4H10![]() |
Neopentane, C5H12![]() |
Unsaturated and alicyclic hydrocarbons | Unsaturated hydrocarbon chains and hydrocarbon rings | 1,3-Butadiene, C4H6![]() |
Cyclohexane, C6H12![]() |
Alkylated benzenes | Six-carbon, aromatic rings with hydrocarbon chain substituent groups | Toluene, C7H8![]() |
Ethylbenzene, C8H10![]() |
Polycyclic aromatic hydrocarbons and related compounds | Multiple, six-carbon aromatic rings, sometimes in combination with other hydrocarbon ring structures | Benzo(a)pyrene, C20H12![]() |
Acenaphthene, C12H10![]() |
Some hydrocarbons are derived from petroleum distillates; others (e.g., pine oil and turpentine) are derived from wood. Many hydrocarbons are used as fuel components, including methane, benzene, toluene, ethylbenzene, and xylenes. One specific group of hydrocarbons currently of interest to exposure assessors are the polycyclic aromatic hydrocarbonspolycyclic aromatic hydrocarbonsGroup of organic chemicals. (See Toxicity Profiles https://19january2021snapshot.epa.gov/region5superfund/ecology/html/toxprofiles.htm#pahs for additional information.) (PAHs), which contain rings of carbon atoms with alternating single and double bonds between carbons.
PAHs are found in coal tar and crude oil, and are sometimes used in medicines or the production of dyes, plastics, and pesticides (ATSDR, 1990).
Commercial production of PAHs is minimal; most PAHs found in the environment are created as a byproduct of incomplete combustion of fossil fuels or wood. PAHs can be created through burning of any organic material, including smoking cigarettes and grilling meat, vegetables, or other foods.
Some PAHs occur as byproducts of industrial processes such as metal production and refining. PAHs can also be released through natural processes such as volcanic eruptions or forest fires. As PAHs are accidentally produced and released rather than intentionally, they generally occur in mixtures (ATSDR, 1990).
Physicochemical Properties
In general, hydrocarbon rings without alternating single and double bonds and hydrocarbon chains are referred to as alicyclic or aliphatic hydrocarbons, respectively, and hydrocarbon rings with alternating single and double bonds are referred to as aromatic hydrocarbons. The number of double versus single bonds determines whether aliphatic hydrocarbons are "saturated" with respect to hydrogen or "unsaturated" due to the presence of double bonds.
Saturated hydrocarbons are more reactive than unsaturated hydrocarbons and are more easily broken down. The bond pattern in the ringed structure of PAHs, on the other hand, results in a chemical property called aromaticity, which confers stability in these compounds.
The table below provides a summary of key physicochemical factors that are likely to affect partitioning and fate of select aliphatic and aromatic hydrocarbons in the environment. For chemical-specific values, consult the resources provided in the introduction to this module.
Property | Fate and Transport Implications |
---|---|
Aliphatic Hydrocarbons | |
Vapor pressure at 25°C (atm) |
Vapor pressure of aliphatic hydrocarbons increases with decreasing chain length, suggesting that low-molecular-weight hydrocarbons will volatilize readily from the pure organic state, while high-molecular-weight compounds will exhibit low volatility. |
Henry’s Law Constant |
H increases with increasing molecular size of the hydrocarbon, however most non-aromatic hydrocarbons have a Henry’s Law Constant around 10 or greater. The high constant indicates a propensity to volatilize, implying that the compounds will readily move from water to air. |
Solubility in water (mg/L) |
As the number of carbons in the chain increase (i.e., molecular weight increases), solubility tends to decrease; branching also decreases solubility. Aliphatic hydrocarbons are less soluble than aromatic hydrocarbons of similar molecular weight. As hydrocarbons commonly occur in mixtures, solubility can vary greatly depending on the composition of the released hydrocarbon fraction. |
Octanol-Water Partition Coefficient (log value) |
The hydrophobicity of aliphatic hydrocarbons generally increases with increasing chain length; larger hydrocarbons have higher sorption potential and are more likely to bioaccumulate. |
Octanol-Air Partition Coefficient (log value) |
Like most other properties, Koa also increases with increased molecular volume, implying that the high-molecular weight aliphatic hydrocarbons (longer chain length) are less likely to partition to air, and more likely to remain sorbed to soil, sediment, or biota than the shorter-chain hydrocarbons. |
Summary: Lighter aliphatic hydrocarbons—with smaller chain lengths—have higher volatility and water solubility, and are less likely to sorb to particles or sediment than larger, longer-chain hydrocarbons. The behavior of a hydrocarbon mixture will therefore vary greatly depending on the mixture composition, and will change over time as the lighter constituents will more readily volatilize and migrate through soils, while heavier constituents will settle into soils, sorb to organic matter, and persist at the site of release. | |
Aromatic Hydrocarbons | |
Vapor pressure at 25°C (atm) |
Vapor pressure is inversely related to PAH size. PAHs with more aromatic rings have higher vapor pressures and are more likely to sorb to particulate matter in air than smaller PAHs. |
Henry’s Law Constant |
Henry’s Law Constants decrease with increasing molecular weight of PAHs, suggesting that low-molecular weight PAHs volatize readily from water to air whereas higher molecular weight PAHs will remain in water. |
Solubility in water (mg/L) |
PAHs have low water solubility, meaning they are primarily found sorbed to particles suspended in the water column or settled to sediment. |
Octanol-Water Partition Coefficient (log value) |
High Kow values signify an affinity to move from water to lipid, suggesting potential for bioaccumulation and bioconcentration in aquatic systems. |
Octanol-Air Partition Coefficient (log value) |
High log Koa values imply that PAHs in the atmosphere will sorb to particulates |
Summary: While air release of PAHs is most likely, residence time in air is limited. PAHs are expected to partition to soil or sediment. |
Routes
Exposure to hydrocarbons occurs most commonly from unintentional ingestion or inhalation. Exposure from dermal contact can also occur.
Route | Potential Sources of Hydrocarbon Exposure |
---|---|
Inhalation |
|
Ingestion |
Ingestion of hydrocarbons occurs as the compounds are a common contaminant in processed foods, particularly foods that are grilled or smoked. PAHs can also occur in non-processed foods such as fruits and vegetables that are grown in environments with contaminated soil or air.
|
Dermal Contact |
Dermal exposure can occur through contact with contaminated soil or water, or anthropogenic surfaces such as asphalt roads or coal tar surfaces.
|
Note: The Routes Tool Set of EPA ExpoBox provides additional information and resources organized by route.
Media
Hydrocarbons are common contaminants in multiple environmental media, including air, water, and soil.
Media | Sources of Hydrocarbons |
---|---|
Air |
|
Water |
|
Soil |
The main source of hydrocarbons in soils is atmospheric deposition.
|
Consumer Products |
Hydrocarbons are found in many consumer products (e.g., gasoline and other fuels, furniture polish, household cleansers, and propellants). |
Note: The Media Tool Set of EPA ExpoBox provides additional information and resources organized by media.
Exposed Populations
Hydrocarbons, especially PAHs, are ubiquitous in the environment and the general population can be exposed in both indoor and outdoor environments. Certain populations are at risk for higher exposures than the general population:
- Cigarette smokers or people who live or work in close proximity to smokers may be exposed to PAHs through inhalation of smoke.
- As PAHs are a common contaminant of vehicle exhaust, urban populations may be more highly exposed than rural populations; however, rural populations may have high exposures to diesel fuels used in farm equipment or trucks, which might be less efficient at combustion due to older age than some vehicles in urban areas.
- Certain occupations may result in high exposure to PAHs, including but not limited to: mechanics, construction workers involved with pavement, roads, or roofs, utility workers exposed to creosote, coal miners or those in the petroleum refinery business, steel foundry and aluminum workers.
Note: The Lifestages and Populations Tool Set of EPA ExpoBox has addition information about particular population groups and lifestages.
Tools
Perfluorinated Compounds (PFCs)
Perfluorinated Compounds (PFCs) contain a carbon chain with fluorine atoms attached in the place of hydrogen atoms and one or more atom or functional groups attached to the end. These compounds are synthetic and used in a variety of manufacturing and industrial applications, including fire resistance and non-stick surfaces. A wide variety of consumer products contain varying levels of PFCs.
These chemicals have been used for many decades in products that resist or repel oil, grease, and water-based liquids. These include products advertised as stain resistant or non-stick, such as cookware, carpets, upholstered furniture, or other fabrics, as well as non-advertised products that have repellent qualities, such as microwave popcorn bags and food packaging.
Example PFC Structures | ||
---|---|---|
8:2 Fluorotelomer Alcohol (8:2 FTOH)![]() |
Perfluorooctanoic acid (PFOA)![]() |
Perfluorooctanesulfonic acid (PFOS)![]() |
Physicochemical Properties
PFCs are generally resistant to further degradation in the environment or within biota (Olsen et al., 2007; Harada et al., 2004). The carbon-fluorine bonds that characterize PFCs are very strong and stable in air at high temperatures; are not readily degradable by strong acids, alkalis, or oxidizing agents; and generally do not undergo photolysis (Lau et al., 2007).
In general, the atmospheric lifespan of long-chain PFCs (LCPFCs) (i.e., 8 or more carbons) is on the order of days to weeks under most atmospheric conditions (Lau et al., 2007). The compounds tend to partition into other environmental media. The half-lives in soil of the C6 through C11 alkylates range from about 1 to 3 years and increase with increasing chain length (Washington et al., 2010). Based on the physicochemical characteristics of these compounds, the half-lives of LCPFCs and precursors in water also are expected to be very long.
The table below provides a summary of key physicochemical factors that are likely to affect partitioning and fate of select PFCs in the environment. For chemical-specific values, consult the resources provided in the Chemical Class Overview.
Property | Fate and Transport Implications |
---|---|
Vapor pressure at 25°C (atm) |
Vapor pressure varies depending on the alkyl chain-length of the PFC. Low vapor pressure of some PFCs contributes to the phenomenon of Long-Range Atmopsheric Transport (LRAT). PFCs remain in the vapor state for extended periods and move readily through the atmosphere, resulting in PFC contamination in areas far from the source of release. |
Solubility in water (mg/L) |
While PFCs are lipid soluble, they are also moderately water-soluble. Many PFCs are acids that will dissociate in freshwater, which increases their solubility. This allows them to remain in the water column. |
Octanol-Water Partition Coefficient (log value) |
PFCs are specifically designed to be hydrophobic and oleophobic, making it difficult to determine a Kow value. However, biomonitoring studies have indicated a tendency to partition into organic fractions (biota), where they bind to proteins in blood serum rather than partition to fats. PFCs with longer alkyl chains are more likely to bioaccumulate and biomagnify than those with shorter chains. |
Summary: PFCs are a unique type of compound in that they are both hydrophobic and oleophobic. They can be measured in the water column, but will also bioaccumulate in biota. They move readily out of products into the air, and can spread long distances through the atmosphere due to their low vapor pressure, meaning exposure can occur far from the source. |
Routes
Ingestion is the primary pathway identified for human exposures to PFCs, but exposures from inhalation and dermal contact can also occur.
Route | Potential Sources of PFC Exposure |
---|---|
Inhalation |
|
Ingestion |
|
Dermal Contact |
Although exposure from dermal contact to PFCs is not considered a dominant route of exposure, people could be exposed via uptake from carpets or other surfaces such as clothing. |
Note: The Routes Tool Set of EPA ExpoBox provides additional information and resources organized by route.
Media
LCPFCs and precursors can be released to various environment media through multiple scenarios. PFCs can be released from manufacturing sites or from use of consumer or industrial products, and secondary releases at sites such as waste water treatment plants (WWTPs) and landfills are possible. These releases generally result in PFCs directly entering water or air, however, release to soils and sediment is also possible, particularly for secondary release scenarios such as application of biosolids to agricultural fields.
Media | Sources of PFCs |
---|---|
Air |
|
Water and Sediment |
|
Soil |
|
Indoor Dust |
|
Note: The Media Tool Set of EPA ExpoBox provides additional information and resources organized by media.
Exposed Populations
PFCs may be found in consumer products, household products, and food packaging, resulting in exposure to the general population. However, some populations may be at risk of higher exposure levels:
- PFCs are persistent in the environment and may be subject to long-range atmospheric transport, resulting in higher environmental contamination levels in certain regions. Therefore, people who live in certain regions, may be at higher risk of exposure.
- The lipophilic nature of PFCs may contribute to the accumulation of these chemicals in breast milk. Infants who are breastfed may be exposed to the chemicals through ingestion. Additionally, in utero exposure can occur due to transfer through cord blood. Infant food and formula have also been shown to contain PFCs, probably due to migration from food packaging.
- Because PFCs bioaccumulate, individuals who consume high amounts of fish or aquatic mammals (i.e., seal, whale), may be exposed to high levels of PFCs, particularly if the fish is sourced from contaminated waters. Fatty cuts of beef or other meats may also contain measureable PFC contamination.
- Residents who live near certain industrial sites may be at risk of exposure through contaminated drinking water, soil, or air.
Note: The Lifestages and Populations Tool Set of EPA ExpoBox has addition information about particular population groups and lifestages.
Tools
Phthalates and Bisphenol A (BPA)
Phthalates contain a phenyl ring with two attached and extended acetate groups. Many of these compounds are liquids used to make plastics more flexible and resilient, called plasticizers. Low-molecular-weight phthalates (e.g., DMP, DEP, DBP, DIPB) may be found in some personal-hygiene and cosmetic products (NRC, 2008). High-molecular-weight phthalates (e.g., DEHP, DOP, and DINP) are used in plastic tubing, food packaging and processing materials, containers, vinyl toys, vinyl floor coverings, and building products. Di(2-ethylhexyl) phthalate (DEHP) is the most widely used phthalate.
Bisphenol A (BPA), while not a phthalate, is similar to phthalates in that it is mainly used to make polycarbonate plastic and epoxy resins, and is used in food and beverage packaging. BPA is also used in thermal printing applications such as cash register receipts as a chemical dye developer. While the major use of this high production volume chemical is polycarbonate plastics and expoxy resins, its use in food packaging and receipts represent significant human exposure routes (U.S. EPA, 2012).
Example Phthalate and BPA Structures | ||
---|---|---|
General Phthalate Structure![]() |
Bis(2-ethylhexyl)phthalate (DEHP)![]() |
Bisphenol A (BPA)![]() |
Physicochemical Properties
The table below provides a summary of key physicochemical factors that are likely to affect partitioning and fate of select phthalates in the environment. For chemical-specific values, consult the resources provided in the Chemical Class Overview.
Property | Fate and Transport Implications |
---|---|
Vapor pressure at 25°C (atm) |
Low volatility of most phthalates means that they are not likely to move from water to air, residence time in air is limited. Low volatility is exploited by uses of phthalates in plastic products intended for high-heat conditions, however, phthalates have been shown to volatilize at appreciable amounts from products such as paints, upholstery, and flooring, because of the high surface-area-to-volume ratio of these uses. |
Henry’s Law Constant |
Phthalates have low Henry’s Law Constants, which correspond with their low vapor pressures and implies a tendency to favor partitioning to water over air. Henry’s Law Constant increases with increased size of the phthalate alkyl chain length, implying that larger molecular weight phthalates have a higher evaporative potential. |
Solubility in water (mg/L) |
Phthalates have low water solubility, however, phthalates are so widely used that even with low solubility they can be detected at significant concentrations in urban runoff, surface water, and drinking water. |
Octanol-Water Partition Coefficient (log value) |
High Kow values imply that phthalates are hydrophobic, and preferentially partition to biota, soils, or sediments as opposed to remaining in the water column. This implies likelihood to bioaccumulate and biomagnify in animals and humans. Kow increases with increasing alkyl chain length, meaning larger phthalates are more hydrophobic. |
Octanol-Air Partition Coefficient (log value) |
High Koa values imply that phthalates are likely to sorb to dust or other particles in the air and settle, rather than remain in a volatile state. |
Summary: Phthalates are more likely to be found in water or soil than in air. Phthalates that are released into the air or volatilized will sorb to particles and settle into dust; similarly phthalates released into water are likely to sorb to organic matter or bioaccumulate in biota. Larger-chained phthalates have lower solubility in air and water than the shorter-chained phthalates. Despite this, phthalates can still be measured in air because the use patterns result in high levels of release to air. |
Routes
Humans may be exposed to phthalates via ingestion, inhalation, and dermal contact for their whole lifetime, including in utero (Latini, 2005).
BPA exposure can occur through direct contact with products that use BPA, such as plastics, receipts, or other thermal papers. Residual BPA may be found in recycled paper products due to recycling of thermal papers. BPA may also be found in foods. Canned foods can become contaminated with BPA because it is used in the can lining. BPA can also leach from plastic containers into food or drink, especially if microwaved or if the plastic has been degraded by high heat.
Note: The Routes Tool Set of EPA ExpoBox provides additional information and resources organized by route.
Media
Phthalates and BPA may occur as contaminants in most environmental media.
Media | Sources of Phthalates and BPA |
---|---|
Air |
|
Water |
|
Soil |
|
Food |
|
Consumer Products |
|
Note: The Media Tool Set of EPA ExpoBox provides additional information and resources organized by media.
Exposed Populations
Phthalates and BPA are ubiquitous in consumer and household products, as they are used in personal care products and many types of plastics. Therefore, the general population may be exposed to phthalates and BPA through a number of different scenarios:
- Exposures to phthalates can occur through the use of some medications or medical devices (Wittassek et al., 2011). This exposed population may be of particular concern depending on the medical conditions for which they are using the phthalate-containing devices or taking the phthalate-containing medications.
- Additionally, some research has shown that women have higher phthalate exposure than men, due to the number of personal care products (soaps, shampoos, lotions, cosmetics) that contain phthalates and the market’s predominance by women (James-Todd et al., 2012).
- Children may also experience higher exposures than adults, due to the presence of phthalates in plastic children’s toys and bottles and the tendency for children to put items in their mouth more frequently than adults. In fact, many products specifically intended for children to put in their mouths (i.e., teething toys, pacifiers) are made from soft plastics containing phthalates.
- Workers in occupations dealing with receipts, such as cashiers and restaurant servers, may have higher exposures to BPA than the general public.
Note: The Lifestages and Populations Tool Set of EPA ExpoBox has addition information about particular population groups and lifestages including workers, children, and women of child-bearing age.
Tools
Volatile Organic Compounds (VOCs)
Volatile organic compounds (VOCs) are a subcategory of organic compounds with a high vapor pressure at room temperature. Because VOC is a category based on a physicochemical property, individual VOCs are also classified as hydrocarbons, pesticides, or other organic compounds.
Many of the compounds addressed elsewhere in this module and the Pesticides Module are VOCs, including DDT, chlordane, phthalates, polycyclic aromatic hydrocarbons (PAHs), polychlorinated biphenyls (PCBs), and several others. VOCs can be further categorized by their degree of volatility into three subgroups:
- Very volatile organic compounds (VVOCs
VVOCsFor example, propane and butane. Boiling point between <0 to 50–100 °C. See reference: https://19january2021snapshot.epa.gov/iaq/voc2.html#classifications), such as butane and propane;
- Volatile organic compounds (VOCs
VOCsBoiling point between 50–100 to 240–260 °C. See reference: https://19january2021snapshot.epa.gov/iaq/voc2.html#classifications), such as acetone, toluene, benzene, tetrachloroethylene [PCE], trichloroethylene [TCE], vinyl chloride, and xylene; and
- Semivolatile organic compounds (SVOCs
SVOCsFor example, DDT, phthalates, and fire retardants. Boiling point between 240–260 to 380–400 °C. See reference: https://19january2021snapshot.epa.gov/iaq/voc2.html#classifications), such as pesticides (e.g., DDT, chlordane), phthalates, polycyclic aromatic hydrocarbons (PAHs), and polychlorinated biphenyls (PCBs).
High volatility means that air is the most important environmental media for this group of compounds, and inhalation is likely to be the exposure route of concern. Indoor environments are of particular interest because VOCs tend to off-gas from consumer products, cleaning products, and building materials like wall paint, upholstery, and flooring.
The general population may be exposed to VOCs through every-day products and materials. Certain populations may be at risk for higher exposure due to activities that result in higher VOC emissions:
- Many household cleaning products release VOCs. People with professions as cleaners (i.e., maids, janitors) or members of the household responsible for cleaning may have higher exposure to VOCs.
- Paints, gasoline, and pesticides can release VOCs. Additionally, many new building supplies, such as carpeting, rugs, or new furniture, can release VOCs. Construction workers, painters, and landscapers may have high occupational exposure to VOCs.
Persistent Organic Pollutants (POPs)
Persistent organic pollutants (POPs), which are also referred to as persistent, bioaccumulative, and toxic compounds (PBTs, or sometimes just PB chemicals), are a subcategory of organic compounds that are highly persistent due to their physical and chemical properties.
The Stockholm Convention on Persistent Organic Pollutants (POPs) is a global treaty to eliminate or reduce the release of POPs into the environment. In the Stockholm Convention, POPs are defined as organic compounds that become widely distributed throughout the environment and remain intact for long periods of time, accumulate in the fatty tissue of living organisms and are found at higher concentrations at higher trophic levels, and are toxic to humans and wildlife. There are currently 22 POPs listed in the Stockholm Convention, including those included in the initial convention in 2001 or new ones added during subsequent meetings in 2009, and 2011.
Substance | Category |
---|---|
Aldrin | Pesticide |
Chlordane | Pesticide |
DDT | Pesticide |
Dieldrin | Pesticide |
Endrin | Pesticide |
Heptachlor | Pesticide |
Hexachlorobenzene | Pesticide, Industrial Chemical, Byproduct |
Mirex | Pesticide |
Toxaphene | Pesticide |
Polychlorinated biphenyls (PCBs) | Industrial Chemical, Byproduct |
Polychlorinated dibenzo-p-dioxins (PCDD) | Byproduct |
Polychlorinated dibenzofurans (PCDF) | Byproduct |
Alpha hexachlorocyclohexane | Pesticide, Byproduct |
Beta hexachlorocyclohexane | Pesticide, Byproduct |
Chlordecone | Pesticide |
Hexabromobiphenyl | Industrial Chemical |
Hexabromodiphenyl ether and heptabromodiphenyl ether (commercial octabromodiphenyl ether) | Industrial Chemical |
Lindane | Pesticide |
Pentachlorobenzene | Pesticide, Industrial Chemical, Byproduct |
Perfluorooctane sulfonic acid, its salts and perfluorooctane sulfonyl fluoride | Industrial Chemical |
Technical endosulfan and its related isomers | Pesticide |
Tetrabromodiphenyl ether and pentabromodiphenyl ether (commercial pentabromodiphenyl ether) | Industrial Chemical |
Source: Stockholm Convention Exit
Both organic and inorganic compounds can be persistent, bioaccumulative, and toxic (or any combination of the three). U.S. EPA (1999) defines these terms as follows:
- Persistent compounds have a transformation half-life of > 2 months
- Bioaccumulative compounds have a fish bioaccumulation factor or bioconcentration factor of ≥ 1,000
- Toxic compounds are identified using accepted toxicity studies
A substance’s persistence is of interest when assessing exposures:
- Some persistent compounds have the potential for long-range transport from sources. For example, elemental mercury emitted to the atmosphere as a vapor can remain in the atmosphere for over a year. This period of time allows the mercury to be transported great distances from the point of emissions by weather patterns before it is deposited to soil, lakes, or other surfaces.
- Some persistent compounds also have the potential to pose exposures many years after they are released to the environment. For example, PCBs are degraded very slowly in the environment; as a result, a spill of PCBs (such as from an electric transformer) can result in elevated levels of PCBs in the soil at that location for years (or even decades) to come.
A substance’s ability to bioaccumulate is also relevant to exposure assessment, especially in combination with longer persistence:
- When a substance that bioaccumulates is also persistent in an organism (and therefore is not metabolized or degraded rapidly), concentrations of that substance can increase over time and may result in greater potential for exposure.
- Substances that bioaccumulate can also result in exposure through the food chain. For example, dioxins and methyl mercury in an aquatic ecosystem can be absorbed by algae, which are then consumed by small fish, which subsequently can be consumed by larger fish.
- If the levels of a persistent and bioaccumulative substance increase with successive levels of a food chain, the process is sometimes referred to as biomagnification. In this case, organisms at the top of a food chain will experience exposures that are greater than if they were only exposed directly to the chemical in the environment.
- Persistent and bioaccumulative substances may be of concern to humans if people consume contaminated organisms from the top of the food chain, such as large, piscivorous fish.
General Tools
Below are tools that are generally applicable to assessing exposure to organic compounds.
References
ATSDR (Agency for Toxic Substances and Disease Registry). (1990). Toxicological Profile for Polycyclic Aromatic Hydrocarbons (PAHs). Atlanta, GA: U.S. Department of Health and Human Services, Public Health Service.
ATSDR (Agency for Toxic Substances and Disease Registry). (2000). Toxicological Profile for Polychlorinated Biphenyls (PCBs). Atlanta, GA: U.S. Department of Health and Human Services, Public Health Service.
Boulanger, B; Vargo, J; Schnoor, JL; Hornbuckle, KC. (2004). Detection of Perfluorooctane Surfactants in Great Lakes Water Exit. Environ Sci Technol 38: 4064-4070.
Davis, KL; Aucoin, MD; Larsen, BS; Kaiser, MA; Hartten, AS. (2007). Transport of Ammonium Perfluorooctanoate in Environmental Media near a Fluoropolymer Manufacturing Facility Exit. Chemosphere 67: 2011-2019.
Egeghy, PP; Lorber, M. (2011). An Assessment of the Exposure of Americans to Perfluorooctane Sulfonate: A Comparison of Estimated Intake with Values Inferred from NHANES Data Exit. J Expo Sci Environ Epidemiol 21: 150-168.
Harada, K; Saito, N; Inoue, K; Yoshinaga, T; Watanabe, T; Sasaki, S; Kamiyama, S; Koizumi, A. (2004). The influence of time, sex and geographic factors on levels of perfluorooctane sulfonate and perfluorooctanoate in human serum over the last 25 years. J Occup Health 46: 141-147.
James-Todd, T; Stahlhut, R; Meeker, JD; Powell, SG; Hauser, R; Huang, T; Rich-Edwards, J. (2012). Urinary Phthalate Metabolite Concentrations and Diabetes among Women in the National Health and Nutrition Examination Survey (NHANES) 2001-2008. Environ Health Perspect 120: 1307-1313 Exit.
Latini, G. (2005). Monitoring Phthalate Exposure in Humans [Review] Exit. Clin Chim Acta 361: 20-29.
Lau, C; Anitole, K; Hodes, C; Lai, D; Pfahles-Hutchens, A; Seed, J. (2007). Perfluoroalkyl Acids: A Review of Monitoring and Toxicological Findings [Review] Exit. Toxicol Sci 99: 366-394.
Nakayama, SF; Strynar, MJ; Reiner, JL; Delinsky, AD; Lindstrom, AB. (2010). Determination of Perfluorinated Compounds in the Upper Mississippi River Basin. Environ Sci Technol 44: 4103-4109.
NRC (National Research Council). (2008). Phthalates and Cumulative Risk Assessment: The Task Ahead Exit. Washington, DC: National Academies Press.
Olsen, GW; Burris, JM; Ehresman, DJ; Froehlich, JW; Seacat, AM; Butenhoff, JL; Zobel, LR. (2007). Half-Life of Serum Elimination of Perfluorooctanesulfonate, Perfluorohexanesulfonate, and Perfluorooctanoate in Retired Fluorochemical Production Workers Exit. Environ Health Perspect 115: 1298-1305.
Saito, N; Harada, K; Inoue, K; Sasaki, K; Yoshinaga, T; Koizumi, A. (2004). Perfluorooctanoate and perfluorooctane sulfonate concentrations in surface water in Japan. J Occup Health 46: 49-59.
Sinclair, E; Kannan, K. (2006). Mass Loading and Fate of Perfluoroalkyl Surfactants in Wastewater Treatment Plants Exit. Environ Sci Technol 40: 1408-1414.
Trudel, D; Horowitz, L; Wormuth, M; Scheringer, M; Cousins, IT; Hungerbuhler, K. (2008). Estimating Consumer Exposure to PFOS and PFOA Exit. Risk Anal 28: 251-269.
U.S. EPA (U.S. Environmental Protection Agency). (2010). An Exposure Assessment of Polybrominated Diphenyl Ethers (PBDE) (Final). (EPA/600/R-08/086F). Washington, DC.
U.S. EPA (U.S. Environmental Protection Agency). (2012). Partnership to Evaluate Alternatives to Bisphenol A in Thermal Paper. Washington, DC.
Van der Veen, I; de Boer, J. (2012). Phosphorus Flame Retardants: Properties, Production, Environmental Occurrence, Toxicity and Analysis [Review] Exit. Chemosphere 88: 1119-1153.
Washington, JW; Yoo, H; Ellington, JJ; Jenkins, TM; Libelo, EL. (2010). Concentrations, Distribution, and Persistence of Perfluoroalkylates in Sludge-Applied Soils near Decatur, Alabama, USA Exit. Environ Sci Technol 44: 8390-8396.
Wittassek, M; Koch, HM; Angerer, J; Brüning, T. (2011). Assessing Exposure to Phthalates - The Human Biomonitoring Approach [Review] Exit. Mol Nutr Food Res 55: 7-31.