Physical Habitat
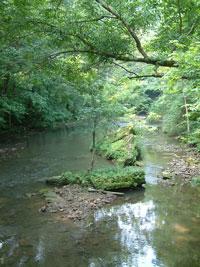
On this Page
- Stream size and channel dimensions
- Channel gradient
- Channel substrate size and type
- Habitat complexity and cover
- Vegetation cover and structure in the riparian zone
- Channel-riparian interactions
Habitat includes all the physical, chemical and biological attributes that affect or sustain the organisms within an ecosystem. However, this section deals with only the structural attributes of habitat (Kaufmann et al. 1993, 1999). Physical habitat does not include physicochemical streamwater attributes (e.g., water chemistry, temperature, clarity, quantity and light intensity). It includes geomorphologic characteristics and biological attributes that determine habitat structure and affect energy inputs.
Figure 2. Channelized tributary to Little Miami River, Ohio. Shallow root structure is insufficient to stabilize stream channel and artificial stream path alters gradient and development of habitat complexity.The difference between relatively unimpaired physical habitat for aquatic life (see Figure 1) and degraded habitat (see Figure 2) can be aesthetically obvious. However, we recommend that in all cases the six attributes listed be considered before deciding to include or defer physical habitat as a candidate cause.
Physical habitat attributes vary naturally with physiographic and climatic setting and watershed size. Thus, anthropogenic alterations of these attributes from conditions expected naturally for a stream (given its physiography, climate and size) represent the stressor gradient for stream biota. Having a clear idea about what is natural given the region, lithology, soil type and climate is particularly important when evaluating physical habitat.
Most natural streams are characterized by sequences of faster riffle and slower pool habitats that provide diverse conditions (e.g., cover, hydraulics, substrates) to meet life history requirements of organisms. For example, large fish may require deep pools to provide refugia during low flow periods, and both small and large fish require refuges from predators. Lithophilic spawning fish and sprawling macroinvertebrates require unembedded interstitial spaces within stable, coarse gravel, cobble or boulder substrata.
Exceptions to this pattern most often occur in regions with low stream gradients, where bedrock may be buried by unconsolidated sands or silts. For example, streams in the Mississippi Alluvial and Southeast USA Coastal Plains ecoregions may be sand-bottomed with little or no coarse inorganic substrates. Most coarse substrates in these streams are woody debris (Wallace and Benke 1984).
The conceptual diagram (Figure 3) shows how sources and activities lead to altered physical habitat and subsequently to biological effects. If you are still deciding what aspects of physical habitat are of greatest interest, we recommend reading through the conceptual diagram and this entire page. If you already know the attributes of interest, you may skip to Ways to Measure to review how these attributes may be measured.
Stream size and channel dimensions strongly define the potential amount of habitat available to organisms (Kaufmann et al. 1999). Within a region, the general size class of a stream in terms of its drainage area, stream order and annual runoff is relatively consistent. However, these relationships can vary among climatic regions with differing precipitation and evapotranspiration regimes.
Anthropogenic activities can alter channel dimensions by changing the frequency of high discharges (i.e., bankfull or floods) or the discharge at low flow. However, flow alteration is also treated as a distinct candidate cause (see the Flow Alteration module).
Channel gradient (slope or downstream elevation change of the stream bed) determines the channel's potential energy and water's velocity and ability to move bed and bank material. Stream power, the capacity of a stream to move materials, is a function of channel gradient, cross-sectional area, shape, hydraulic roughness and discharge. Channel gradient interacts with these variables to influence the microhabitat diversity within the stream reach, particularly viawater velocity.
Moreover, these hydraulic characteristics interact with bed particle size to affect stream bed stability (Kaufmann et al. 1999). Generally, channel gradient is determined by the geomorphologic setting of a stream. However, anthropogenic activities that change bedload, or one of the other factors affecting stream power, can alter channel gradients. In addition, anthropogenic activities such as channelization may directly alter the effective channel gradient.
Channel substrate size and type determine habitat variety and overall habitable surface area for epilithic and interstitial aquatic animals (Pugsley and Hynes 1986). For benthic organisms, substrate size and packing influence stability for attachment in varying flows (Biggs et al. 1997, Downes et al. 1997, Matthaei et al. 2000). This includes not only characteristics of geological substrates forming the stream bottom, but also presence of aquatic macrophytes or other organic materials (e.g., woody debris).
Substrate size and bedform (e.g., the presence of riffles or pools) influence hydraulic roughness of the bed and variation in current velocities in a channel. Substrate size and sorting (variation in particle sizes) also affect the size of spaces within substrates that provide cover for smaller fish, salamanders and macroinvertebrates.
Human activities that disturb stream banks or increase upland erosion increase sediment supply to stream channels. Increases in fine sediments decrease mean substrate size and increase the percentage of fine sediments. Suspended and fine bedded sediments are treated as a distinct candidate cause (see the Sediments module). Increases in bedload can destabilize channels, leading to channel aggradation and accelerate channel migration.
- Extent and distribution of geomorphological units, such as pools and riffles
- Presence, size and amount of large woody debris
- Presence, extent and diversity of other in-channel cover (e.g., undercut banks, boulders, macrophytes, algae)
- Residual pool depth and channel complexity
- Channel sinuosity
Geomorphologic units (i.e., pool-riffle, step-pool sequences), channel sinuosity and hydraulic roughness result from and contribute to processes that dissipate flowing water's energy in stream channels. Woody debris and living plants along stream banks also contribute to this dissipation of energy.
For water column-dwelling fishes, pools or other low-velocity areas (e.g., eddies, backwaters) are flow refugia (Negishi et al. 2002). Pools also act as refuges when water depths become too low elsewhere in the channel at low discharges. Residual pool depth is a measure of the pool depth that would be remaining if discharge were reduced to 0.
For many organisms, riffles represent turbulent, highly oxygenated stream areas with coarse sediments that resist movement during both normal and high flows (Matthaei et al. 1999, 2000). Channel complexity suggests a mixture of both low-velocity and high-velocity habitats and contributes to the diversity of organisms residing in a stream (Jenkins et al. 1984, Tockner et al. 2003).
Any type of in-channel cover or water depths that shields fishes or macroinvertebrates from predators or competitors acts as refuge from these biotic interactions.
Vegetation in the riparian zone plays many important roles in streams. Most of these roles are considered in other modules. This is because the riparian zone generally affects the delivery of stressors to the stream channel, but is not the point of exposure for stream organisms.
Riparian woody vegetation is a primary source of woody debris in the channel. Riparian vegetation shades streams and provides leaf litter, an important food resource for stream organisms. Riparian vegetation stabilizes stream banks, supplies cover and benthic substrates along the channel edge and increases habitat complexity (Sweeney 1992, Trimble 1997, Sweeney et al. 2004). When flows exceed bankfull discharge, vegetation on the floodplain contributes to hydraulic roughness and moderates erosion (see Temperature module).
Channel-riparian interactions play an important—but variable—role in stream structure and function. Depending on the stream setting and hydrographic regime, natural overbank flows may only be periodic (≈ 1 per year) and inundate small areas of the adjacent riparian zone. Overbank flows may also be characterized by more infrequent flooding of large portions of the floodplain or seasonal inundation of adjacent riparian wetlands.
Overbank flows during periods of high discharge are important for stream geomorphology. They can transport inorganic and organic materials between the channel and its floodplain. Anthropogenic alterations along stream banks (floodwalls, levees, channelization) or upstream (dams) are intended to contain flooding but also change natural stream processes.
When describing altered physical habitat as a candidate cause, anthropogenic stream channel alterations (e.g., rip-rap, bank armoring structures, culverts) are obvious. They alter flow, erosion and deposition patterns, resulting in habitat alteration. If a stream segment is a concrete channel or a reservoir, that attribute can be listed as a candidate cause. Anthropogenic alterations in the riparian zone or broader catchment also may be sources or indicators of potential physical habitat changes.
Checklist of Sources, Site Evidence and Biological Effects
This module addresses changes in physical habitat as proximate stressors. Candidate causes associated with altered physical habitat should be considered when observations support portions of the source-to-impairment pathways (Figure 3). The conceptual diagram and some of the other information also are useful for Step 3: Evaluate Data from the Case.
Below is a checklist of observations to help determine if altered physical habitat should be included as a candidate cause. The list can guide you in collecting evidence to support, weaken or eliminate physical habitat as a candidate cause, but it is not exhaustive. For more information on specific entries, go to the When to List tab.
Figure 3. A simple conceptual diagram illustrating causal pathways, from sources to impairments, related to physical habitat. Click on the diagram to view a larger version.Consider listing candidate causes associated with altered physical habitat when the following sources, site evidence and biological effects are present:
Sources and Activities
- Presence of a low-head dam or weir downstream that submerges channel features
- Presence of levees, walls or other structures that confine high flows to channel
- Residential, commercial or urban development that increases impervious surfaces
- Agricultural activities designed to move water quickly off the land (e.g., drainage ditches or swales, tile drainage)
- Removal or alteration of riparian vegetation
- Activities that directly alter the channel (e.g., channelization, dredging, pipeline crossings and gravel mining)
- Presence of a dam or weir upstream
- Historical presence of a dam
- Historical or current activities that directly disturb benthic substrates (e.g., dredge mining)
Site Evidence
- Concrete structures, rip-rap or other revetments lining the channel
- Coarse substrates (i.e., boulder, cobble and woody debris) embedded by finer substrates (i.e., sand, silt and clay)
- Streamflow passing between abutments of a bridge or through a culvert or pipe
- Straightening or deepening (i.e., channelization) of stream channel; materials previously removed from the channel mounded adjacent to channel
- Predominance of runs, glides or pools instead of alternating pools and riffles or other fast-water habitats
- Presence of bare, eroded banks lacking vegetation
- Lack or alteration of riparian vegetation
- Head-cut and incision of stream channel
- Extreme width:depth ratios
- Lack of other habitat-forming features (e.g., filamentous algae, aquatic macrophytes, large woody debris, brush and small woody debris, overhanging vegetation, undercut banks or artificial structures)
- Trampling of stream banks and bank vegetation by livestock
Biological Effects
- Reduced abundance of sunfish or bass (Centrarchidae) or salmon, trout or whitefishes (Salmonidae)
- Reduced abundance of piscivorous fishes
- Reduced abundance of darters (Etheostoma, Percina), sculpins (Cottus), or madtoms (Noturus)
- Reduced abundance of young-of-year fishes
- Reduced fish taxonomic richness
- Reduced relative abundance of clinging and sprawling invertebrates
- Changes in relative abundance of invertebrate functional feeding groups (e.g., grazers)
- Changes in periphyton biomass and species composition
- Sediments
- Flow
- Temperature
- Dissolved oxygen
On this Page
- Sources and Activities that Suggest Listing Candidate Causes Associated with Altered Physical Habitat
- Site Evidence that Suggests Listing Candidate Causes Associated with Altered Physical Habitat
- Biological Effects that Suggest Listing Candidate Causes Associated with Altered Physical Habitat
- Site Evidence that Supports Exclusion of Candidate Causes Associated with Altered Physical Habitat as a Candidate Cause
Sources and Activities that Suggest Listing Candidate Causes Associated with Altered Physical Habitat
Channelization
The most obvious sources or activities associated with altered physical habitat are direct alterations of the stream channel. Dredging operations to straighten and deepen stream channels (i.e., channelization) are seldom observed directly. Observations that may indicate channelization include sinuosity near one (where sinuosity equals the distance along the channel thalweg between two points divided by the straight line distance between those two points); low wetted width to thalweg depth ratio; rectangular channel cross-section; dredged sediments piled along and adjacent to the channel; or dominance of run or glide habitats.
The thalweg is the deepest water depth in a channel cross-section, usually at the center of water flow within a channel. Runs or glides are channel areas characterized by non-turbulent flow over a relatively flat stream bottom (Platts et al. 1983). Water depths are intermediate between riffles and pools. In glides, the surface water gradient is nearly level and current velocities are slower than in runs. However, particularly in the case of alluvial channels, there may be rapid and ongoing channel dimension adjustments. This can include degradation (lowering) or aggradation (raising) of the streambed and mass wasting of stream banks, which can proceed both upstream or downstream of the channelized reach (Simon 1989).
Historically, channelization was conducted to drain lands to increase farming and construction area or for flood control. As a result, small stream channels were lost and drainage density in many watersheds has decreased (Meyer and Wallace 2001). Drainage density is the ratio of the total channel length in a drainage basin to the basin area (km/km2).
In urban areas, stream channels may be buried in culverts or pipes or entirely replaced by stormwater drainage systems (Elmore and Kaushal 2008). This habitat degradation is so severe that such channels are not typically considered biologically relevant nor identified as impaired aquatic systems.
Dams
A dam either upstream or downstream of a stream reach may alter physical habitat. Effects on the channel are obvious when a downstream dam impounds a reservoir. They may not be as obvious with smaller run-of-the-river type dams or weirs (Poff and Hart 2002). Upstream of reservoirs, dam operation to manage pool heights and water releases may periodically inundate tributary streams. Run-of-the-river dams or weirs, including many smaller, older dams, generally increase thalweg depths for some distance upstream and create pool habitat. However, the water remains mostly within the stream channel at normal flows.
There are estimated to be over one million dams with heights less than four meters throughout the United States (Poff and Hart 2002). Dams with significant hydraulic residence times (i.e., the length of time water remains in a reservoir) will capture bedload and suspended sediments and disrupt sediment transport (Kondolf 1997, Poff and Hart 2002). As a result, water released from the dam has energy to move sediment but has little or no sediment load. This “hungry water” (Kondolf 1997) erodes the channel bed and banks downstream of the dam, resulting in streambed incision and coarsening of bed materials. This continues at least until a new equilibrium state is reached, with the bed becoming armored and flows no longer able to move remaining bed materials (Ligon et al. 1995).
After a dam is breached, some former slackwater sediment deposits will be transported downstream and deposited over coarser substrates (Orr et al. 2008). Deposits upstream of the dam may be incised by the stream to form steep, easily-eroded banks of fine sediments. They can produce the incised, meandering gravel-bed streams observed in the Northern Piedmont ecoregion of the eastern United States (Walter and Merritts 2008). This reinterpretation of the historical effects of mill dams may apply to other regions with historical construction of small, run-of-the river dams or weirs.
Mining
Another channel alteration is caused by instream mining for sand and gravel used as construction aggregate (Kondolf 1997). Gravel mining alters channel geometry and bed elevation. It also may include clearing of vegetation from sandbars and riparian zones and digging of deep pits in the channel. The result is disruption of natural channel morphology and production of local sediment deficits. In some cases, a headcut is produced by gravel mining. This can result in channel incision, bed coarsening and lateral channel instability, which can migrate both upstream and downstream from the actual mining site (Kondolf 1997).
Other types of mining also can locally alter stream channels. Mountaintop removal mining for coal completely buries headwater stream channels with valley fills (Meyer and Wallace 2001, Palmer et al. 2010). Surface subsidence associated with long-wall coal mining can reduce stream gradients and alter stream flows (PADEP 1999). Deposition of iron and other metal hydroxides downstream from acidic mine drainage sources can embed and cement stream sediments. Dredge mining of placers in stream channels can alter thalweg profiles and reduce residual pool lengths and maximum pool depths (Mossop and Bradford 2006).
Impervious Surfaces
Often, more widespread alterations of stream physical habitat result from anthropogenic activities in the watershed that increase stormflow magnitudes and frequencies. Residential, commercial and urban development increase impervious surface area. Some agricultural activities (e.g., drainage ditches and tile drainage systems) route stormwater quickly off of agricultural fields. These activities increase soil erosion and input of fine sediments to streams, but particularly increase peak discharges, which erode stream banks (Chin 2006). This results in stream widening, fine sediment deposition in pools and substrate embeddedness.
Site Evidence that Suggests Listing Candidate Causes Associated with Altered Physical Habitat
Several anthropogenic activities directly alter the stream channel and affect its physical habitats. The most obvious evidence is the presence of rip-rap, gabions (i.e., usually wire mesh cages containing rocks) or concrete structures (e.g., walls and other revetments) lining the channel. These structures typically are used to prevent lateral movement of the active channel and limit land loss and damage to infrastructure (e.g., roads). These structures usually affect stream banks, which are important transition zones within the riparian ecotone.
The stream bank is the part of the stream channel between the bed's lateral margins when normally wetted at baseflow and at bankfull height, where flood flows begin to spread laterally over the floodplain (Florsheim et al. 2008). Riparian vegetation on the stream bank interacts more with the wetted channel. Construction of artificial structures removes this vegetation and its functions (Rhodes and Hubert 1991, Wood and Sites 2002, Sweeney 1993). Such structures also may increase flood velocities along stream banks and prevent reestablishment of riparian vegetation, arrest exchanges of sediment between channel and bank, and destroy habitat for fauna that use riparian zones. Moreover, the design of these structures often fails to account for bank erosion processes or consider the appropriate scale for bank erosion management or secondary, long-term or cumulative effects (Piégay et al. 2005, Florsheim et al. 2008).
Other localized structures that can alter stream channels include bridge abutments, culverts or pipes that constrict flows, particularly higher flows. Constricted flows below bridges and culverts erode the stream bed and banks (Langendoen 2000, Johnson et al. 2002). Moreover, pipes or culverts usually lack benthic sediments and may act as migration barriers for aquatic fauna (Warren and Pardew 1998, Poplar-Jeffers et al. 2009). This barrier effect is enhanced when a pipe or culvert is placed above the stream channel and flows erode a scour pool below its outlet.
Figure 4. Examples of headcuts on Hotophia Creek and Big Creek, Mississippi, respectively.
Photos courtesy of F.D. Shields and S. Knight, USDA, ARS, National Sedimentation Laboratory, Oxford, MSThe presence of a headcut is another indicator of stream channel degradation. A headcut is an abrupt change in stream bed elevation marking the upstream migration of channel incision in a stream (see Figure 4). Development of a headcut can result from gully development associated with poor agricultural erosion control or channelization of streams (Shields et al. 1998). In some cases, the channel incision associated with such headcuts may eliminate stream-floodplain interactions.
Logging of riparian forests may have reduced the size of woody debris falling into many forested stream ecosystems. This is particularly true if the riparian zone is no longer forested, but also occurs in streams where the riparian forest has been allowed to regenerate. Initially logging may increase large woody debris stocks, but long-term loadings decrease due to increased decay and transport rates and reduced inputs (Chen et al. 2005).
Large woody debris can be important to the geomorphology of streams (Flebbe and Dolloff 1995, Abbe and Montgomery 1996, Hassan et al. 2005). The size of the woody debris relative to channel width is an important factor affecting the movement of the wood during high discharges (Berg et al. 1998). Smaller woody debris and brush can be used for cover by fish and invertebrates (Harvey et al. 2005), but such cover is more ephemeral.
Beyond noting the obvious types of evidence discussed so far, it is also helpful to examine the channel's dimensions and classify it by geomorphological type. Channel dimensions include wetted width, thalweg depth (and their ratio) and bankfull channel width, depth and their ratio. A high ratio of bankfull channel width to depth indicates stream bank degradation or stream bed aggradation, while a low ratio may indicate stream bed entrenchment. Rosgen (1996) presents a classification system to describe channel geomorphological types and assess stream channel condition.
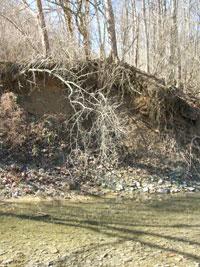
- Low ratio of stream bank height to bankfull height (i.e., usually less than one)
- Low ratio of riparian vegetation rooting depth to stream bank height (i.e., usually much less than one)
- Low rooting density of plants (i.e., stream bank relatively lacking in rooted vegetation)
- Stream bank composed of relatively fine materials, such as fine gravels, sand or clay
- Steep stream bank angle or slope (i.e., near to 90%)
- Stream bank material either stratified (i.e., showing layers of sediment) or containing soil lenses (i.e., buried soil layers)
- Lack of stream bank surface protection by debris or vegetation (absence of vegetation or other material) that armor the stream bank
Other structures in the stream channel are also important for stream biota. These structures supply cover or form habitat, and the lack of such cover or habitat-forming features is an indicator of poor habitat quality. These features include woody debris, filamentous algae, aquatic macrophytes, overhanging vegetation, undercut banks or artificial structures that supply cover (Jakober et al. 1998, Lyons et al. 2000, Roni et al. 2002, Fritz et al. 2004). One needs to evaluate these on a case-by-case basis. For example, too much cover by filamentous algae or aquatic macrophytes may indicate impairment.
Biological Effects that Suggest Listing Candidate Causes Associated with Altered Physical Habitat
We encourage consideration of local fauna and flora as indicators of habitat disturbance. Physical habitat is closely linked to many other stressors, and biological effects usually are not sufficiently specific to be symptomatic of impaired physical habitat alone. However, certain biological effects (e.g., fish, macroinvertebrate and algae assemblage changes) often are associated with physical habitat degradation.
Changes in Fish Assemblages
Changes in substrate or cover within a stream may provide less suitable habitat for shelter or reproduction (Matthews 1998). Fishes that rely on natural wood in streams for cover will be adversely affected by decreased inputs or removal of wood (e.g., Centrarchidae, Salmonidae) (Angermeier and Karr 1984). Diverse and abundant submerged structures tend to increase habitat, and thus fish diversity (Barbour et al. 1999).
Fish species that seek cover in streamside vegetation may be adversely affected by its loss or alteration. Fishes that rely on floodplain connections for reproduction and nurseries may be affected if floodplain access is restricted (Lasne et al. 2007). If channels become incised, large pool-dwelling fishes (e.g., top carnivores or piscivores such as Centrarchidae) may be favored while fishes that require shallow riffles [e.g., darters (Etheostoma, Percina), sculpins (Cottus) or madtoms (Noturus)] or stream-margin habitats (e.g., young-of-year of Salmonidae, Centrarchidae, Cyprinidae or Catostomatidae) may decline (Moore and Gregory 1988, Aadland 1993, LaVoie and Hubert 1996).
Dams and reservoirs may drastically alter fish assemblages. Upstream, reservoirs provide habitat for facultative riverine species (e.g., Dorosoma cepedianum and Cyprinus carpio) that require lentic reproductive habitats. They also fragment the stream system for obligate river species that migrate during the year. These migratory species include salmonids in Pacific Northwest rivers; Atlantic sturgeon (Acipenser oxyrhynchus), Alabama shad (Alosa alabamae) and mountain mullet (Agonostomus monticola) in southern Atlantic and Gulf Coast rivers; and Atlantic shad (Alosa sapidissima) in mid-Atlantic rivers (Pringle 1997).
Changes in Macroinvertebrate Assemblages
Macroinvertebrate assemblages reflect stream habitat complexity (Brown 2007). Channel alteration resulting from channelization, other direct modifications of stream channels, incision or channel widening generally reduces habitat complexity.The abundance of habitat specialists with specific flow and substrate requirements also declines. For example, the relative abundance of clingers (invertebrates with behavioral or morphological adaptations for living attached to hard surfaces) may decrease in stream reaches with homogeneous substrate composition, current velocity and water depth (Rabeni et al. 2005).
In sandy, lowland streams or rivers that otherwise lack stable substrates, submerged large woody debris and snags may support high invertebrate productivity. Collector-filterers (e.g., Hydropsychidae) that feed on fine particulate organic matter suspended in the water column (Benke et al. 1984) may be especially abundant on large woody debris. In other streams, woody debris locally alters stream depth, gradient and substrate, affecting adjoining microhabitats. This may change the abundance and biomass of functional feeding groups (e.g., decreasing collector-filterers and grazers, increasing collector-gatherers and predators and changing dominant shredder groups) (Wallace et al. 1995).
Bank alterations may reduce the amount of streamside habitat available for invertebrate species (Rankin 1989). Floodplain connections are also important for invertebrate productivity (Benke 2001). If organic matter inputs and retention in a stream are reduced due to loss of riparian vegetation or channel incision, the invertebrates that rely on this food source (i.e., detritivores, such as shredders and collector-gatherers) will decline in abundance. This in turn can affect invertebrate food resources for fish.
Macroinvertebrate traits (e.g., maximum size, body form, feeding habit, mode of reproduction, lifespan, dispersal strategies) are related to habitat variables, particularly hydraulics. For example, Lamoroux et al. (2004) found the percentage of macroinvertebrates with maximum body size greater than 20 mm was inversely correlated with hydraulic force and substrate roughness. In contrast, the percentage of macroinvertebates with maximum body size between 5 and 10 mm was positively correlated with the same variables. Other macroinvertebrate traits positively correlated with hydraulic force and substrate roughness included streamlined body form, lifespan less than 1 year, univoltine reproductive cycle, egg masses cemented or fixed to substrates, aerial active dispersal, respiration through the exoskeleton, a temporarily or permanently attached life style and scraper feeding habitats. Beyond these measurements of channel hydraulics, this paper suggests that trait metrics could be sensitive measures of assemblage responses to physical habitat alterations.
Changes in algae assemblages
Figure 6. An incised channel, Bobo Bayou, Mississippi.
Courtesy of F.D. Shields, USDA, ARS, National Sedimentation Laboratory, Oxford, MSLarge inorganic substrates or wood in streams provide stable surfaces for algae, a food source for invertebrates. Factors affecting the stability of such substrates can affect algal standing crops (Myers et al. 2007), and loss of these surfaces reduces the area available for algae growth. Channel incision (see Figure 6) may reduce floodplain connections area for algal growth and nutrient retention (Coleman and Dahm 1990). Hill et al. (2003) showed that algal changes were related to substrate size, canopy cover, stream width, channel slope and the proximity-weighted sum of riparian disturbance. Algae also respond to the pattern of riffles and pools (Stevenson 1996) by influencing shear stress and delivery of nutrients to periphytic assemblages.
Site Evidence that Supports Exclusion of Candidate Causes Associated with Altered Physical Habitat as a Candidate Cause
General advice on excluding candidate causes from your initial list is provided in Step 2 of the Step-by-Step Guide and in Tips for Listing Candidate Causes.
Exclusion of altered physical habitat as a candidate cause should be based on high quality in-stream measurements and the absence of evidence of sources or activities that alter stream physical habitats. However, few watersheds across the United States have not experienced at least some human activities that disturb the dynamic quasi-equilibrium of stream channels (Vannote et al. 1980, Thrush et al. 2000). This includes the historical elimination of beaver, which were once a keystone species in many stream ecosystems across the northern United States (Naiman et al. 1988). You could demonstrate that the identified impairment does not occur at reference sites with similar physical habitat. Therefore, excluding physical habitat usually requires at least a screening-level assessment.
Site observations or measurements may at least support deferring consideration of physical habitat to focus on other candidate causes. For example, physical habitat is unlikely to be a cause of impairment if the stream channel has alternating riffle-pool sequences, is not incised and lacks apparent physical alteration. Similarly, if stream banks and the riparian zone lack erosion scars and are well-vegetated with plants native to and characteristic of the ecoregion, physical habitat is unlikely to be a cause. For alluvial channels, a more detailed description of good physical habitat may be found in Thrush et al. (2000).
There are diverse measurements that may be used to quantify stream physical habitat. A variety of publications are available that describe methods for measuring physical habitat in streams. Some of these publications are focused on fish habitat (Bain and Stevenson 1999) or have a state or regional focus (Simonson et al. 1994, Overton et al. 1997, Somerville and Pruit 2004, Doll et al. 2003).
Two general procedures, intended to be applicable nationwide, are available. Field methods for these procedures involve thorough collection of quantitative data representative of a stream reach. The documents outline methods used to measure and summarize physical habitat variables in the U.S. Environmental Protection Agency's Environmental Monitoring and Assessment Program (Kaufmann and Robison 1998, Kaufmann et al. 1999) and the U.S. Geological Survey's National Ambient Water Quality Assessment program (Fitzpatrick et al. 1998).
Physical Habitat Attributes | Measurements |
---|---|
Stream size and channel dimensions | Stream order Stream catchment or watershed area (km2) Thalweg depth (cm) Wetted width (m) Cross-sectional area (i.e., wetted width x thalweg depth) (m2) Wetted width : thalweg depth ratio Bank angle (degrees) Bank undercut distance (m) Bankfull width (m) Bankfull height (m) |
Channel gradient | Water surface gradient (m/m or %) |
Channel substrate size and type | Mean substrate size class Mean substrate diameter (mm) Substrate embeddedness (%) Percent substrate, specific size classes: bedrock (>4000 mm) boulder (250-4000 mm) cobble (64-250 mm) coarse gravel (16-64 mm) fine gravel (2-16 mm) sand (0.6-2 mm) fines (silt/clay, <0.6 mm) Percent substrate, coarse (>16 mm) Percent substrate, sand + fines (<2 mm) Percent substrate, wood Percent substrate, organic detritus Percent substrate, concrete Percent substrate, hard pan Relative bed stability |
Habitat complexity and cover | Percent, specific habitat types: falls cascades riffle run or glide impoundment pool plunge pool lateral scour pool trench pool backwater pool dry channel subsurface flow Percent fast-water habitats (falls + cascades + rapids + riffles) Percent slow-water habitats (glides + all pool types) Percent pool (all pool types) Channel sinuosity Residual pool vertical profile area (m2) Residual pool volume (m3) Count of residual pools with residual depth >50 cm, >75 cm, >100 cm Residual pool length (m) Residual pool area (m2) Residual pool depth (m) Percent of pool volume filled with sediment fines Areal cover, specific cover types (proportion) filamentous algae aquatic macrophytes large woody debris brush and small woody debris overhanging vegetation boulder and rock ledges undercut banks artificial structures Areal cover, all types except algae and macrophytes (m2 or %) Areal cover, large types (i.e., large wood, boulders, undercut banks, artificial structures) (m2 or %) Areal cover, natural types (i.e., large wood, brush, overhanging vegetation, boulders and undercut banks) (m2 or %) Large woody debris within active channel (i.e., submerged) [count or volume (m3)] Large woody debris above active channel (i.e., not submerged) [count or volume (m3)] |
Vegetation cover and structure in the riparian zone | Percent, canopy density Riparian canopy cover (proportion) Riparian mid-layer cover (proportion) Riparian ground-layer cover (proportion) Riparian bare ground (proportion) |
Anthropogenic alterations | Riparian human disturbance, specific types buildings pavement roads pipes parks or lawns row-crop agriculture pasture or grass fields logging mining trash revetments culverts or bridge abutments |
Channel-riparian interactions | Incision height (m) |
Qualitative visual assessments that score habitat characteristics on some scale (often from 0 to 20) and combine scores into an index also are widely used. Examples include U.S. EPA's Rapid Assessment Protocol Habitat Assessment (Barbour et al. 1999) and Ohio EPA's Qualitative Habitat Evaluation Index (QHEI) (Rankin 1989). In high gradient streams, the Rapid Habitat Assessment Protocol scores 10 habitat characteristics: epifaunal substrate and available cover, embeddedness, velocity and depth regime, sediment deposition, channel flow status, channel alteration, frequency of riffles or bends, bank stability, bank vegetative protection and riparian vegetative zone width (Barbour et al. 1999). The QHEI scores six habitat characteristics: substrate (type and quality), instream cover (type and quality), channel quality (sinuosity, development, channelization, and stability), riparian/erosion (width, floodplain quality, and bank erosion), pool/riffle (maximum depth, current available, pool morphology, riffle/run depth, riffle substrate stability and substrate embeddedness) and gradient.
While such qualitative assessments may be more rapid, direct measurements or estimates of physical habitat attributes more clearly define habitat alterations at a site. Moreover, Bauer and Ralph (1999) found that measurements based on visual judgments were the least precise habitat variables. They noted that percent pool has relatively low precision, because it is visually estimated and dependent on stream flow at the time of the observation. However, it should also be noted that some measurements listed in the preceding table also rely on visual estimates.
On this Page
About Conceptual Diagrams
Simple Conceptual Model Diagram
Physical habitat alterations, defined here as changes in the structural geomorphic or vegetative features of stream channels, can adversely affect aquatic organisms. Figure 3. A simple conceptual diagram illustrating causal pathways, from sources to impairments, related to physical habitat. Click on the diagram to view a larger version.
Many human activities and land uses can lead to changes in in-stream physical habitat. Mining and resource extraction, agriculture, forestry and silviculture, urbanization and industry can contribute to increased sedimentation and changes in discharge patterns, as well as decreases in stream bank habitat and instream cover, including large woody debris.
Direct alteration of streams channels also can influence physical habitat, by changing discharge patterns, changing hydraulic conditions (water velocities and depths), creating barriers to movement, and decreasing riparian habitat. These changes can alter the structure of stream geomorphological units (e.g., by increasing the prevalence of run habitats, decreasing riffle habitats and increasing or decreasing pool habitats).
Typically, physical habitat degradation results from reduced habitat availability (e.g., decreased snag habitat, decreased riffle habitat) or reduced habitat quality (e.g., increased fine sediment cover). Decreases in habitat availability or habitat quality may contribute to decreased condition, altered behavior, increased mortality or decreased reproductive success of aquatic organisms. Ultimately, these effects may result in changes in population and community structure and ecosystem function.
Detailed Conceptual Model Diagram
Alterations of physical habitat, defined here as changes in the structural geomorphic or vegetative features of stream channels, can adversely affect aquatic organisms, potentially changing community structure and ecosystem function.
This conceptual diagram illustrates linkages between physical habitat-related stressors (middle of diagram), the human activities and sources that can increase those stressors (top of diagram), and the biological responses that can result (bottom of diagram). In some cases, additional steps leading from sources to stressors, modes of action leading from stressors to responses and other modifying factors also are shown. The narrative generally follows the diagram top to bottom, left to right.
Linking Sources to Stressors
Many human activities and land uses can lead to changes in in-stream physical habitat. Activities that decrease riparian, bank, or watershed vegetation (e.g., mining, agriculture, forestry, urbanization) and the specific sources associated with those activities (e.g., mines, cropfields, pastures, impervious surfaces) can affect physical habitat by increasing bank, channel, and watershed erosion; reducing cover, bank habitat, and large woody debris; and increasing stormwater runoff.
Bank and channel erosion also may occur when livestock have access to riparian areas and streams and trample banks. Erosion can result in increased bedload and deposited sediment in streams (see the Sediment module for more information on sediment-related stressors), though this relationship often depends upon the geology and the gradient of the system.
Direct alteration of stream channels also influences physical habitat. Instream sand and gravel mines can alter sediment particle size distributions within streams. Impoundments, detention basins, levees, road crossings and stream channelization, armoring, burial, piping, and dredging can adversely affect in-stream habitat by changing discharge patterns, changing hydraulic conditions (water velocities and depths), creating barriers to movement, increasing bank and channel erosion, and decreasing riparian connectivity and habitat.
Stormwater runoff, point effluent discharges, and drainage infrastructure (e.g., tile drains) also can alter discharge patterns. All of these sources associated with channel alteration can alter the structure of stream geomorphological units (e.g., by increasing the prevalence of run habitats, decreasing riffle habitats, and increasing or decreasing pool habitats). In addition, these sources may increase channel incision, decrease channel sinuosity, and alter channel width-to-depth ratios.
Linking Stressors to Biological Responses
The activities and sources discussed above can result in decreased physical habitat availability (e.g., decreased snag habitat, decreased riffle habitat) or decreased physical habitat quality (e.g., increased fine sediment cover). Decreases in habitat availability or habitat quality may contribute to decreased condition, altered behavior, increased mortality, or decreased reproductive success of aquatic organisms.
Ultimately, these effects may result in changes in population and community structure and ecosystem function. Changes in population and community structure that may be associated with changes in physical habitat include: decreases in riparian- and floodplain-dependent taxa; decreases in top carnivores, piscivores, darters, salmonids and young-of-year fish; decreases in clinging macroinvertebrates; decreases in taxa richness or diversity; and changes in functional feeding groups. Ecosystem function also can be affected directly.
This section presents an annotated bibliography of references providing information on stressor-response relationships for physical habitat, as well as other general background information. This is not meant to be a comprehensive bibliography of references dealing with physical habitat. Rather, it is meant to highlight a few references that may be especially useful.
You may need a PDF reader to view some of the files on this page. See EPA’s About PDF page to learn more.
- Allan JD (2004) Landscapes and riverscapes: the influence of land use on stream ecosystems. Annual Review of Ecology and Systematics 35:257-284.
This paper describes the relationships between changes in land use and in-stream habitat quality. It reviews the influence of scale on these relationships.
- Bain MB, Stevenson NJ (Eds.) (1999) Aquatic Habitat Assessment: Common Methods. American Fisheries Society, Bethesda MD.
This book reviews the common methods used to assess aquatic habitats. It includes aquatic habitats other than streams and also covers a wider range of habitat features than this module.
- Bauer SB, Ralph SC (2001) Strengthening the use of aquatic habitat indicators in Clean Water Act programs. Fisheries 26:14-25.
This review argues that in-stream habitat quality should be more explicitly integrated into water quality standards under the Clean Water Act.
- Bednarek AT (2001) Undamming rivers: a review of the ecological impacts of dam removal. Environmental Management 27:803-814.
This paper reviews the impacts of dam removals on physical habitats in streams and the larger scale effects on stream communities.
- Bunn SE, Arthington AH (2002) Basic principles and ecological consequences of altered flow regimes for aquatic biodiversity. Environmental Management 30(4):492-507.
This article covers the effects of flow alteration on physical habitat and aquatic biota. Influence of flow alteration leading to physical habitat alteration is described for fish, invertebrates, and aquatic plants. Consequences of converting lotic systems to lentic are described. Life history of aquatic species is related to flow along with impacts on populations from disruption of stream connectivity. Lastly, stream flow is connected to ability of invasive and exotic species to successfully invade.
- Cunjak RA (1996) Winter habitat of selected stream fishes and potential impacts from land-use activity. Canadian Journal of Fisheries and Aquatic Sciences 53(Supplement 1):267-282.
This paper reviews the winter habitat requirements of stream fishes with emphasis on salmonids. It further discusses how this habitat is affected by land use in the stream catchments with reference to impacts from water withdrawal, altered flow regimes, erosion and sedimentation.
- Detenbeck NE, DeVore PW, Niemi GJ, Lima A (1992) Recovery of temperate-stream fish communities from disturbance: a review of case studies and synthesis of theory. Environmental Management 16:33-53.
This paper reviews cases of recovery of fish communities for 49 temperate streams was monitored following an anthropogenic disturbance. The review found that the fish communities were not resilient to long-term disturbances that included alteration of physical habitats in the absence of some mitigation effort.
- Fitzpatrick FA, Waite, IR, D'Arconte PJ, Meador MR, Maupin MA, Gurtz ME (1998) Revised Methods for Characterizing Stream Habitat in the National Water-Quality Assessment Program. U.S. Geological Survey, Raleigh NC. Water Resources Investigations Report 98-4052.
This document outlines the methods used to measure and summarize the physical habitat variables in the National Water-quality Assessment Program of the U.S. Geological Survey.
- Hart DD, Finelli CM (1999) Physical-biological coupling in streams: the pervasive effects of flow on benthic organisms. Annual Review of Ecology and Systematics 30:363-395.
The paper reviews the effects of flow at the habitat level on different ecological processes for benthic macroinvertebrates. These processes include dispersal, predator-prey interactions, habitat use, competition and resource acquisition. The authors conclude that local flow environments are the primary factor affecting habitat use by different benthic organisms.
- Jackson CR, Sturm CA (2002) Woody debris and channel morphology in first- and second-order forested channels in Washington's coast ranges. Water Resources Research 38:1177-1191.
This paper reviews the role of woody debris the geomorphology and ecology of small headwater streams in the Pacific Northwest. The study compared small stream wood frequencies to those in larger streams, evaluated the effects of large woody debris frequency and size on stream morphology, and explored the relationships between landscape and channel habitat in small streams.
- Kaufmann PR, Levine P, Robinson EG, Seeliger C, Peck DV (1999), Quantifying Physical Habitat in Wadeable Streams. U.S. Environmental Protection Agency, Washington DC. EPA/620/R-99/003.
This document outlines methods used to measure and summarize physical habitat variables in the U.S. Environmental Protection Agency's Environmental Monitoring and Assessment Program.
- Mammoliti CS (2002) The effects of small watershed impoundments on native stream fishes: a focus on the Topeka shiner and hornyhead chub. Transactions of the Kansas Academy of Sciences 105:219-231.
Using the Topeka shiner (Notropis topeka) and hornyhead chub (Nocomis biguttatus) as examples, this paper reviews the effects of small impoundments on obligate stream species. The review finds that impoundments reduce the quantity and quality of stream habitat, affect fish reproductive and feeding behavior, and can increase the number and sizes of predator species with a stream system.
- Roni P, Beechie TJ, Bilby RE, Leonetti FE, Pollock MM, Pess GR (2002) A review of stream restoration techniques and a hierarchical strategy for prioritizing restoration in Pacific Northwest watersheds. North American Journal of Fisheries Management 22:1-20.
This paper reviews stream restoration techniques that have been used in the Pacific Northwest in efforts to restore salmonid populations and places them in the context of a hierarchical framework for planning watershed restorations.
- Rosenfeld J (2003) Assessing the habitat requirements of stream fishes: an overview and evaluation of different approaches. Transactions of the American Fisheries Society 132(5):953-968.
This paper reviews different approaches for assessing the habitat requirements of stream fishes. These approaches are different forms of habitat models (e.g., presence/absence, habitat capacity, microhabitat and bioenergetic models).They point out that these habitat models must be created and used within the context of the fish's life history when different life stages have different habitat requirements.
- Wheeler AP, Angermeier PL, Rosenberger AE (2005) Impacts of new highways and subsequent landscape urbanization on stream habitat and biota. Reviews in Fisheries Science 13:141-164.
This paper reviews the short-term and long-term effects of highway crossings on stream habitat and communities, including the effects of urban development associated with road development.
You may need a PDF reader to view some of the files on this page. See EPA’s About PDF page to learn more.
- Aadland LP (1993) Stream habitat types: their fish assemblages and relationship to flow. North American Journal of Fisheries Management 13(4):790-806.
- Abbe TB, Montgomery DR (1996) Large woody debris jams, channel hydraulics and habitat formation in large rivers. Regulated Rivers: Research and Management 12:201-221.
- Allan JD (2004) Landscapes and riverscapes: the influence of land use on stream ecosystems. Annual Review of Ecology and Systematics 35:257-284.
- Angermeier PL, Karr JR (1984) Relationships between woody debris and fish habitat in a small warmwater stream. Transactions of the American Fisheries Society 113:716-726.
- Bain MB, Stevenson NJ (Eds.) (1999) Aquatic Habitat Assessment: Common Methods. American Fisheries Society, Bethesda MD.
- Barbour MT, Gerritsen J, Snyder BD, Stribling JB (1999) Rapid bioassessment protocols for use in wadeable streams and rivers: periphyton, benthic macroinvertebrates, and fish (2nd edition). U.S. Environmental Protection Agency, Office of Water, Washington DC. EPA 841-B-99-002.
- Bauer SB, Ralph SC (2001) Strengthening the use of aquatic habitat indicators in Clean Water Act programs. Fisheries 26:14-25.
- Bednarek AT (2001) Undamming rivers: a review of the ecological impacts of dam removal. Environmental Management 27:803-814.
- Benke AC (2001) Importance of flood regime to invertebrate habitat in an unregulated river-floodplain ecosystem. Journal of the North American Benthological Society 20(2):225-240.
- Benke AC, Van Arsdall TC Jr, Gillespie DM (1984) Invertebrate productivity in a subtropical blackwater river: the importance of habitat and life history. Ecological Monographs 54(1):25-63.
- Berg N, Carlson A, Azuma D (1998) Function and dynamics of woody debris in stream reaches in the central Sierra Nevada, California. Canadian Journal of Fisheries and Aquatic Sciences 55:1807-1820.
- Biggs BJF, Duncan MJ, Francoeur SN, Meyer WD (1997) Physical characterisation of microform bed cluster refugia in 12 headwater streams, New Zealand. New Zealand Journal of Marine and Freshwater Research 31:413-422.
- Brown BL (2007) Habitat heterogeneity and disturbance influence patterns of community temporal variability in a small temperate stream. Hydrobiologia 586:93-106.
- Bunn SE, Arthington AH (2002) Basic principles and ecological consequences of altered flow regimes for aquatic biodiversity. Environmental Management 30(4):492-507.
- Chen X, Wei X, Scherer R (2005) Influence of wildfire and harvest on biomass, carbon pool, and decomposition of large woody debris in forested streams of southern interior British Columbia. Forest Ecology and Management 208:101-114.
- Chin A (2006) Urban transformation of river landscapes in a global context. Geomorphology 79:460-487.
- Coleman RL, Dahm CN (1990) Stream geomorphology: effects on periphyton standing crop and primary production. Journal of the North American Benthological Society 2:293-302.
- Cunjak RA (1996) Winter habitat of selected stream fishes and potential impacts from land-use activity. Canadian Journal of Fisheries and Aquatic Sciences 53(Supplement 1):267-282.
- Detenbeck NE, DeVore PW, Niemi GJ, Lima A (1992) Recovery of temperate-stream fish communities from disturbance: a review of case studies and synthesis of theory. Environmental Management 16:33-53.
- Doll BA, Grabow GL, Hall KR, Halley J, Harman WA, Jennings GD, Wise DE (2003) Stream Restoration: A Natural Channel Design Handbook. North Carolina Stream Restoration Institute, North Carolina State University, Raleigh NC. 128 pp.
- Downes BJ, Glaister A, Lake PS (1997) Spatial variation in the force required to initiate rock movement in 4 upland streams: implications for estimating disturbance frequencies. Journal of the North American Benthological Society 16:203-220.
- Elmore AJ, Kaushal SS (2008) Disappearing headwaters: patterns of stream burial due to urbanization. Frontiers in Ecology and the Environment 6(6):308-312.
- Fitzpatrick FA, Waite, IR, D'Arconte PJ, Meador MR, Maupin MA, Gurtz ME (1998) Revised Methods for Characterizing Stream Habitat in the National Water-Quality Assessment Program. U.S. Geological Survey, Raleigh NC. Water Resources Investigations Report 98-4052.
- Flebbe PA, Dolloff CA (1995) Trout use of woody debris and habitat in Appalachian wilderness streams of North Carolina. North American Journal of Fisheries Management 15:579-590.
- Florsheim JL, Mount JF, Chin A (2008) Bank erosion as a desirable attribute of rivers. BioScience 58:519-529.
- Fritz KM, Gangloff MM, Feminella JW (2004) Habitat modification by the stream macrophyte Justicia americana and its effects on biota. Oecologia 140:388-397.
- Hart DD, Finelli CM (1999) Physical-biological coupling in streams: the pervasive effects of flow on benthic organisms. Annual Review of Ecology and Systematics 30:363-395.
- Harvey BC, White JL, Nakamoto RJ (2005) Habitat-specific biomass, survival, and growth of rainbow trout (Oncorhynchus mykiss) during summer in a small coastal stream. Canadian Journal of Fisheries and Aquatic Sciences 62:650-658.
- Hassan MA, Hogan DL, Bird SA, May CL, Gomi T, Campbell D (2005) Spatial and temporal dynamics of wood in headwater streams of the Pacific Northwest. Journal of the American Water Resources Association 41:899-919.
- Hill BH, Herlihy AT, Kaufmann PR, DeCelles SJ, Vander Borgh MA (2003) Assessment of streams of the eastern United States using a periphyton index of biotic integrity. Ecological Indicators 2:325-338.
- Jackson CR, Sturm CA (2002) Woody debris and channel morphology in first- and second-order forested channels in Washington's coast ranges. Water Resources Research 38:1177-1191.
- Jakober MJ, McMahon TE, Thurow RF, Clancy CG (1998) Role of stream ice on fall and winter movements and habitat use by bull trout and cutthroat trout in Montana headwater streams. Transactions of the American Fisheries Society 127:223-235.
- Jenkins RA, Wade KR, Pugh E (1984) Macroinvertebrate-habitat relationships in the River Teifi catchment and the significance to conservation. Freshwater Biology 14:23-42.
- Johnson PG, Hey RD, Brown ER, Rosgen SL (2002) Stream restoration in the vicinity of bridges. Journal of the American Water Resources Association 38:55-67.
- Kaufmann PR (Ed.) (1993) Physical habitat. in: Hughes RM (Eds). Stream Indicator and Design Workshop. U.S. Environmental Protection Agency, Office of Research and Development, Washington DC. EPA/600/R-93/138.
- Kaufmann PR, Levine P, Robinson EG, Seeliger C, Peck DV (1999) Quantifying Physical Habitat in Wadeable Streams. U.S. Environmental Protection Agency, Washington DC. EPA/620/R-99/003.
- Kaufmann PR, Robison EG (1998) Physical habitat characterization. in: Lazorchak JM, Klemm DJ, Peck DV (Eds)
- Environmental Monitoring and Assessment Program - Surface Waters: Field Operations and Methods for Measuring the Ecological Condition of Wadeable Streams.
- U.S. Environmental Protection Agency, Washington DC. EPA/620/R-94/004F.
- Kondolf GM (1997) Hungry water: effects of dams and gravel mining on river channels. Environmental Management 21:533-551.
- Lamouroux N, Doledec S, Gayraud S (2004) Biological traits of stream macroinvertebrate communities: effects of microhabitat, reach, and basin filters. Journal of the North American Benthological Society 23(3):449-466.
- Langendoen EJ (2000) CONCEPTS: Conservational channel evolution and pollutant transport system. U.S. Department of Agriculture, Agricultural Research Service, National Sedimentation Laboratory, Oxford MS. Research Report No. 16.
- Lasne E, Lek S, Laffaille P (2007) Patterns in fish assemblages in the Loire floodplain: the role of hydrological connectivity and implications for conservation. Biological Conservation 139:258-268.
- LaVoie WJ, Hubert WA (1996) Use of three types of stream-margin habitat by age-0 brown trout late in the growing season. Hydrobiologia 317:89-95.
- Ligon FK, Dietrich WE, Thrush WJ (1995) Downstream ecological effects of dams. BioScience 45:183-192.
- Lyons J, Trimble SW, Paine LK (2000) Grass versus trees: managing riparian areas to benefit streams of central North America. Journal of the American Water Resources Association 36:919-930.
- Mammoliti CS (2002) The effects of small watershed impoundments on native stream fishes: a focus on the Topeka shiner and hornyhead chub. Transactions of the Kansas Academy of Sciences 105:219-231.
- Matthaei CD, Arbuckle CJ, Townsend CR (2000) Stable surface stones as refugia for invertebrates during disturbance in a New Zealand stream. Journal of the North American Benthological Society 19:82-93.
- Matthaei CD, Peacock KA, Townsend CR (1999) Patchy surface stone movement during disturbance in a New Zealand stream and its potential significance for the fauna. Limnology and Oceanography 44:1091-1102.
- Matthews WJ (1998) Patterns in Freshwater Fish Ecology. Chapman and Hall, New York NY.
- Meyer JL, Wallace JB (2001) Lost linkages and lotic ecology: rediscovering small streams. Pp. 295-317 in: Press MC, Huntly NJ, Levin S (Eds). Ecology: Achievement and Challenge. Blackwell Science, Oxford UK.
- Moore KMS, Gregory SV (1988) Response of young-of-the-year cutthroat trout to manipulation of habitat structure in a small stream. Transactions of the American Fisheries Society 117:162-170.
- Mossop B, Bradford MJ (2006) Using thalweg profiling to assess and monitor juvenile salmon (Oncorhynchus spp.) habitat in small streams. Canadian Journal of Fisheries and Aquatic Sciences 63:1515-1525.
- Myers AK, Marcarelli AM, Arp CD, Baker MA, Wurtsbaugh WA (2007) Disruptions of stream sediment size and stability by lakes in mountain watersheds: potential effects on periphyton biomass. Journal of the North American Benthological Society 26:390-400.
- Naiman RJ, Johnston CA, Kelley JC (1988) Alteration of North American streams by beaver. BioScience 38:753-762.
- Negishi JN, Inoue M, Nunokawa M (2002) Effects of channelization on stream habitat in relation to a spate and flow refugia for macroinvertebrates in northern Japan. Freshwater Biology 47:1515-1529.
- Orr CH, Kroiss SJ, Rogers KL, Stanley EH (2008) Downstream benthic responses to small dam removal in a coldwater stream. River Research and Applications 24:804-822.
- Overton CK, Wollrab SP, Roberts BC, Radko MA (1997) R1/R4 (Northern/Intermountain Regions) Fish and Fish Habitat Standard Inventory Procedures Handbook. U.S. Department of Agriculture, Forest Service, Intermountain Research Station, Ogden UT. General Technical Report INT-GTR-346.
- PADEP (1999) The Effects of Subsidence Resulting from Underground Bituminous Coal Mining on Surface Structures and Features and Water Resources. Pennsylvania Department of Environmental Protection, Harrisburg PA.
- Palmer MA, Bernhardt ES, Schlesinger WH, Eshleman KN, Foufoula-Georgiou E, Hendryx MS, Lemly AD, Likens GE, Loucks OL, Power ME, White PS, Wilcock PR (2010) Mountaintop mining consequences. Science 327:148-149.
- Piegay H, Darby SE, Mosselman E, Surian N (2005) A review of techniques available for delimiting the erodible river corridor: a sustainable approach to managing bank erosion. River Research and Applications 21:773-789.
- Platts WS, Megahan WF, Minshall GW (1983) Methods for Evaluating Stream, Riparian, and Biotic Conditions. U.S. Forest Service, Intermountain Forest and Range Experiment Station, Ogden UT. General Technical Report INT-138.
- Poff NL, Hart DD (2002) How dams vary and why it matters for the emerging science of dam removal. BioScience 52:659-668.
- Poplar-Jeffers IO, Petty JT, Anderson JT, Kite SJ, Strager MP, Fortney RH (2009) Culvert replacement and stream habitat restoration: implications from brook trout management in an Appalachian watershed, U.S.A. Restoration Ecology 17(3):404-413.
- Pringle CM (1997) Exploring how disturbance is transmitted upstream: going against the flow. Journal of the North American Benthological Society 16:425-438.
- Pugsley CW, Hynes HBN (1986) Three-dimensional distribution of winter stonefly nymphs, Allocapnia pygmaea, within the substrate of a southern Ontario river. Canadian Journal of Fisheries and Aquatic Sciences 43:1812-1817.
- Rabeni CF, Doisy KE, Zweig LD (2005) Stream invertebrate community functional responses to deposited sediment. Aquatic Sciences 67:395-402.
- Rankin ET (1989) The Qualitative Habitat Evaluation Index (QHEI): Rationale, Methods, and Application. Exit Ohio EPA, Division of Water Quality Planning and Assessment, Ecological Assessment Section, Columbus OH.
- Rhodes HA, Hubert WA (1991) Submerged undercut banks as macroinvertebrate habitat in a subalpine meadow stream. Hydrobiologia 213(2):149-153.
- Roni P, Beechie TJ, Bilby RE, Leonetti FE, Pollock MM, Pess GR (2002) A review of stream restoration techniques and a hierarchical strategy for prioritizing restoration in Pacific Northwest watersheds. North American Journal of Fisheries Management 22:1-20.
- Rosenfeld J (2003) Assessing the habitat requirements of stream fishes: an overview and evaluation of different approaches. Transactions of the American Fisheries Society 132(5):953-968.
- Rosgen DL (1996) Applied River Morphology. Wildland Hydrology, Pagosa Springs CO. 246 pp.
- Shields FD Jr, Knight SS; Cooper CM (1998) Rehabilitation of aquatic habitats in warmwater streams damaged by channel incision in Mississippi. Hydrobiologia 382:63-86.
- Simon A (1989) A model of channel response in disturbed alluvial channels. Earth Surface Processes and Landforms 14:11-26.
- Simonson TD, Lyons J, Kanehl PD (1994) Evaluating fish habitat in Wisconsin streams. U.S. Department of Agriculture, Forest Service, North Central Forest Experiment Station, St. Paul MN. Special Technical Report NC-164.
- Somerville DE, Pruitt BA (2004) Physical Stream Assessment: A Review of Selected Protocols for Use in the Clean Water Act Section 404 Program. Prepared for the U.S. Environmental Protection Agency, Office of Wetlands, Oceans, and Watersheds, Washington DC. 213 pp.
- Stevenson RJ (1996) The stimulation and drag of current. Pp. 321-340 in: Stevenson RJ, Bothwell ML, Lowe RL (Eds). Algal Ecology: Freshwater Benthic Ecosystems. Academic Press, San Diego CA.
- Sweeney BW (1992) Streamside forests and the physical, chemical, and trophic characteristics of piedmont streams in eastern North America. Water Science and Technology 26:2653-2673.
- Sweeney BW (1993) Effects of streamside vegetation on macroinvertebrate communities of White Clay Creek in eastern North America. Proceedings of the Academy of Natural Sciences of Philadelphia 144:291-340.
- Sweeney BW, Bott TL, Jackson JK, Kaplan LA, Newbold JD, Standley LJ, Hession WC, Horwitz RJ (2004) Riparian deforestation, stream narrowing, and loss of stream ecosystem services. Proceedings of the National Academy of Sciences USA 101:14132-14137.
- Thrush WJ, McBain SM, Leopold LB (2000) Attributes of an alluvial river and their relation to water policy and management. Proceedings of the National Academy of Sciences USA 97:11858-11863.
- Tockner K, Ward JV, Arscott DB, Edwards PJ, Kollmann J, Gurnell AM, Petts GE, Maiolini B (2003) The Tagliamento River: a model ecosystem of European importance. Aquatic Sciences 65:239-253.
- Trimble SW (1997) Stream channel erosion and change resulting from riparian forests. Geology 25:467-469.
- Vannote RL, Minshall GW, Cummins KW, Sidel JR, Cushing CE (1980) The river continuum concept. Canadian Journal of Fisheries and Aquatic Sciences 37:130-137.
- Wallace JB, Benke AC (1984) Quantification of wood habitat in subtropical coastal plain streams. Canadian Journal of Fisheries and Aquatic Sciences 41(11):1643-1652.
- Wallace JB, Webster JR, Meyer JL (1995) Influence of log additions on physical and biotic characteristics of a mountain stream. Canadian Journal of Fisheries and Aquatic Sciences 52:2120-2137.
- Walter RC, Merritts DJ (2008) Natural streams and the legacy of water-powered mills. Science 319:299-304.
- Warren ML Jr, Pardew MG (1998) Road crossings as barriers to small-stream fish movement. Transactions of the American Fisheries Society 127:637-644.
- Wheeler AP, Angermeier PL, Rosenberger AE (2005) Impacts of new highways and subsequent landscape urbanization on stream habitat and biota. Reviews in Fisheries Science 13:141-164.
- Wood DL, Sites RW (2002) Submerged rootmats: a mesohabitat harboring a distinct insect community in Ozark streams. Journal of Freshwater Ecology 17(3):431-440.
Contacts: Authors & Contributors